Back to Páramo Flora Home
Text: James L. Luteyn
Photographs: Carmen Ulloa Ulloa and Peter Jørgensen, unless specified.
This "Páramo ecosystem" website is based primarily upon information published in the book Páramos: A Checklist of Plant Diversity, Geographical
Distribution, and Botanical Literature (Luteyn, 1999, Memoirs of the New York Botanical Garden Volume 84).
INTRODUCTION TO THE PARAMO ECOSYSTEM
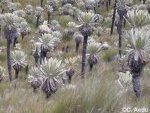 Espeletia |
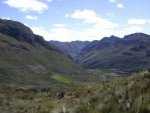 U-shaped valley |
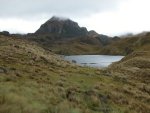 Lake Patoquinoas |
"The páramo zone is the most interesting of the life zones of
the Andes, since it shows to the highest degree, the struggle of plant
and animal life against conditions of extreme cold temperature..."
(Chardón, 1933)
General Definition
Within the tropical regions of Mexico,
Central and South America, Africa, Malesia including New Guinea, and Hawaii,
there is a vegetation type that occurs between the upper limit of continuous,
closed-canopy forest (i.e., forest line or timberline) and the upper limit
of plant life (i.e., snow line) that is characterized by tussock grasses,
large rosette plants, shrubs with evergreen, coriaceous and sclerophyllous
leaves, and cushion plants. This vegetation type is scattered along
the crests of the highest mountain ranges or on isolated mountaintops between
about 3000 meters (m) and 5000 m, like islands in a sea of forest.
Locally these areas are known as "zacatonales" (the Mexican and Guatemalan
volcanic highlands), "páramo" (Central and northern South America),
"jalca" (northern Peru), "puna" (drier areas of the altiplano of the central
Andes), "afroalpine" and "moorland" (East Africa), and "tropical-alpine"
(Malesia).
Although neither Beard (1944), Troll
(1958), nor Lauer (1981) felt the term "alpine" was appropriate for inter-tropical
high altitude landscapes, these vegetation types have been more recently
referred to as "tropical alpine" (Ramsay & Oxley, 1997; Rundel
et al., 1994; Smith & Young, 1987) or "tropicalpine" (Smith &
Cleef, 1988). I do not like to apply the general term "alpine" to
vegetation in the tropics, because it is a term derived from temperate
regions. Furthermore, high elevation tropical climates differ sharply
from those of temperate alpine regions, particularly with respect to seasonality
and diurnal patterns of temperature change (Rundel, 1994). Some authors,
including Walter (1973), Lauer (1981), and Monasterio and Vuilleumier (1986),
advocate using the term páramo in the broadest sense possible, on
a worldwide basis, for all high tropical montane vegetation above the continuous
timberline for the sake of nomenclatural simplicity, if for no other reason.
Monasterio and Vuilleumier would simply add a geographic adjective to characterize
the particular area being discussed, for example, Andean páramo,
African páramo, etc. Andean páramo is often compared
with other alpine and arctic ecosystems (Baruch, 1979; Billings,
1973, 1974, 1979; Billings & Mooney, 1968; Smith &
Young, 1987). For the purposes of this book, the term "páramo"
is used in its regional sense, being restricted to the northern Andes of
South America and adjacent southern Central America. It is here used
as a collective term for the entire landscape unit (or ecosystem) of the
high altitude above continuous forest line and below the perpetual snow
line. Many different plant communities can be found in páramo,
and will be discussed below, but the most widespread are dominated by
tussock-forming grasses.
The word páramo comes from the
Latin word "paramus", according to the Diccionario de la Lengua Española
(Real Academia Española, 1992). Corominas (1973) states that
this Hispanic-Latin word seemingly arose from the mid-western portion of
the northern Iberian Peninsula, but that its exact origin is uncertain.
He further suggests that it may have been adopted by the Romans as a celticism,
or instead is neither Iberian nor Celtic but originates from another Indoeuropean
language in pre-Roman times. In Spain, up until the epoch of the
Conquest, the desertic plateau of arid Castile, which contrasts with the
fertile regions lower down, was called "paramera." Font Quer (1977)
states that in Castilian Spanish the general significance of the word páramo
is a flat plain. The early Spanish explorers applied the word páramo
to north Andean areas that were high, cold, inhospitable, wind- and rainswept,
perhaps reminding them of the plains in their native Iberian Peninsula.
In Colombia, atmospheric moisture in the form of drizzle is often referred
to as "paramitos," while in Ecuador the term "parameando" has come to mean
"it is raining," and in Venezuela one might say "estoy emparamado" when
one is getting wet because of rain and cold (Monasterio, 1980b; Nuñes
& Pérez, 1994; Ramsay, 1992; Vareschi, 1970;
Weber, 1958; pers. comm. with local inhabitants). For definitions
and insight into conceptualizations of the páramo ecosystem by local
"parameros" (inhabitants of the páramos), see López-Zent
(1995) and Zambrano (1993).
The páramo landscape has been
influenced by glaciation. It is irregular and uneven, from
jagged and very rough with erratic rocks to rolling or flat, often times
with many small glacial lakes and tributaries.
It is the source of many of the large rivers of northern South America
(e.g., Río Magdalena and Río Cauca of Colombia, Río
Napo and Río Coca of Ecuador, and Río Orinoco of Colombia
and Venezuela). As will be seen from the discussions in this Introduction,
there is no single definition of páramo, because it is characterized
by a variety of geographic, geologic, climatic, physiognomic, and floristic
features, all of which will be briefly touched upon.
Geographical Distribution
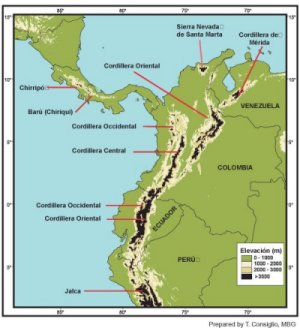 |
In the tropics of the Americas, the páramo
ecosystem is discontinuously distributed between 11°N and 8°S latitudes.
It is concentrated in the northwest corner of South America, mostly in
Venezuela, Colombia, and Ecuador, but there are outliers in Costa Rica,
Panama, and northern Peru (see front and back endpapers, which show in
black the area above 3000 m elevation that is potentially páramo).
The northernmost páramos are located in the Sierra Nevada de Santa
Marta of Colombia, located at about 11°N latitude. The westernmost
páramos are located in Costa Rica, in the Cerro Buena Vista (Cerro
de La Muerte) region of the Cordillera de Talamanca, at about 83°W
longitude. The easternmost páramos are located in north-central
Venezuela, in the state of Lara, at about 70°W longitude. The
southernmost páramos (locally called "jalca") are found in northern
Peru in the department of La Libertad, at about 8°S latitude, just
north of the Cordillera Blanca. Other neotropical areas that have
páramo-like vegetation or that correspond ecologically and have
sometimes been referred to as páramo, but that fall outside the
geographical range or definition used in this book, include Pico Duarte
in the Dominican Republic, the "zacatonales" of Mexico, Pico Naiguatá
(Avila and the Silla de Caracas areas) in the Cordillera Costal of north-central
Venezuela, Pico de la Neblina along the Venezuela/Brazil border, scattered
"humid puna" areas of the eastern slopes of the Peruvian Andes, the "yungas"
region of northeastern Bolivia, scattered areas in Chile and Argentina,
and the Itatiaia area of eastern Brazil.
For other opinions about the geographical
distribution of páramo, see Brack Egg (1986b), Braun (1956), Cleef
(1978, 1981b), Cuatrecasas (1968), Mann (1968), Monasterio (1980b), ONERN
(1976), Ribera et al. (1994), Tosi (1960), Unzueta Q. (1975), Vareschi
(1955), and Vuilleumier and Monasterio (1986).
Climate
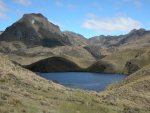 Cloud-free, cold, crisp |
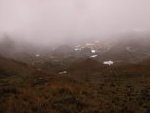 Fog, and drizzle |
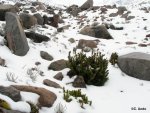 Snow |
The páramos of Colombia and northern
Ecuador are influenced by the intertropical convergence of air masses (a
low pressure trough), because of their geographical location near the Equator.
They are generally humid throughout all or most months of the year with
continuous moisture in the form of rain, clouds, and fog, mostly due to
orographic uplift caused by the Andes. Many páramos receive
more than 2000 mm of rain on their exposed slopes (absolute range 500-ca.
3000 mm/year). They have a high relative humidity averaging 70-85%
(absolute range 25-100%). This contrasts with páramos in the
northernmost Andes of Venezuela, the Sierra Nevada de Santa Marta in northern
Colombia, and in Costa Rica, where there is a marked dry season due to
the influence of the northeast Trade winds (Herrmann, 1970, 1971;
Lauer, 1979a,b). Páramo becomes driest near its southern limits
in southern Ecuador and northern Peru, where they are influenced by two
air masses, one from the Amazon Basin, which has already released its moisture
on the eastern slopes, and another dry cool air mass from the West under
the influence of the Humboldt Current. In addition to the large scale
climatic parameters given above, local microclimates may strongly influence
regional weather patterns (G. Sarmeinto, 1986). In northern Peru,
the páramo environment grades almost imperceptibly into the "puna"
ecosystem, which is more characteristic of the central and southern Andean
altiplano highlands of central Peru, Bolivia, and Argentina. Puna,
in contrast to páramo, is typically xeric with lower humidity, a
shorter wet season, and six months or more of almost no rain. Figure
5 illustrates the overall climate of páramo by the use of climate
diagrams from scattered localities. For additional
climate diagrams,
see Cañadas Cruz (1983), Cleef (1981b), Guhl (1982), Jørgensen
and Ulloa U. (1994), Monasterio and Reyes (1980), Van der Hammen et al.
(1995), and Witte (1994). For comparisons of páramo and puna,
see reviews by Cabrera (1957, 1968, 1976), Lauer (1952), Quintanilla P.
(1983), G. Sarmiento (1986), Troll (1968b), and Young et al. (1997).
Páramos have a generally cold
and humid climate with sudden changes in the weather and a diurnal fluctuation
in temperature from below freezing to as much as 30°C, which often
results in a daily freeze-and-thaw cycle that has been referred to as "summer
every day and winter every night" (O. Hedberg, 1964). During the
dry season, for example, Páramo Piedras Blancas (Venezuela), at
3700-4700 m elevation, shows extremes in air temperatures ranging from
-5°C to -11°C at night to 25-30°C during the day, with freezing
temperatures occurring 325-350 nights of the year (Pérez, 1987a,
1996b; Pfitsch, 1994). Although overall mean annual temperatures
of páramo range from 2°C to 10°C, there is much greater
contrast in the climate of higher elevation areas than is found in lower
zones of the same mountain ranges. Therefore, the environment becomes
harsher and more severe for plant life as altitude increases (Javellas
& Thouret, 1995).
A typical day often begins
cloud-free, cold, crisp, and
occasionally windy until mid morning; then increased cloud
cover from lower elevations, caused by convectional and
orographic uplift, brings rain, sleet, fog, and drizzle for much of the
afternoon; clearing often occurs in the late afternoon or early evening.
Nights are always cold and usually clear with stars filling the skies;
however, frost is frequent in the high páramos and
snow is common
at the highest altitudes. During any given day of the year
rain, ice, snow, and fog may alternate abruptly with clear sunny skies
and elevated temperatures; in one moment the wet cold necessitates
heavy clothing, raincoats, and gloves, while in the next moment, lotion
is needed to protect against sunburn.
For other general references that discuss
páramo climate, see Cuatrecasas (1968), Eidt (1968), Guhl (1974),
Lauer (1981), Monasterio (1980a), Rundel (1994), Schnetter et al. (1976),
J. Snow (1976), Sturm (1978), Sturm and Rangel Ch. (1985), Troll (1968b),
Van der Hammen and Ruiz (1984), Van der Hammen and Santos (1995), Van der
Hammen et al. (1983, 1989, 1995, In press), Weber (1958), and Witte (1994).
Soils
The geology of the Andes is extremely
variable and consequently so are the soils. Most páramo soils
are relatively young and only slightly developed, and are broadly classified
into the orders Andosols, Inceptisols, Histosols, Entisols, and Mollisols
(Buol, et al., 1980; Buringh, 1979; FAO, 1975; Soil Survey
Staff, 1975).
Andosols and Inceptisols include older
soil names such as Ando soils, brown tropical soils, black soils, Onji
soils, humic allophane soils, hydro humic latosols, volcanic soils, and
volcanic ash soils. They are soils formed from and associated with
volcanic ash, have a low supply of (or have lost) bases or iron and aluminum,
and show moderate weathering. In páramo, these may include
Andepts and some Aquepts, as well as Tropepts and Umbrepts. The Andosols
most likely to form in páramos are Aquands, Cryands, and Udands.
Histosols include older soil names such
as bog soils, muck soils, organic soils, and peat soils. They are
soils that are highly organic and are found in very wet places such as
bogs and swamps. These may include Fibrists, Folists, Hemists, and
Saprists.
Entisols are soils that have little
or no evidence of development (i.e., absence of horizons) and have a highly
mineral nature. These are often found near snow line and may include
Aquents, Fluvents, Orthents, and Psamments.
Mollisols are a less common soil group
in the páramo, but are very dark colored and base-rich. The
most likely group to develop are the Aquolls.
At its highest elevations (i.e., superpáramo),
páramo soils are very shallow and coarse with a
high percentage of rock and sand, there is little
to no production of organic matter, and consequently, low water retention.
Superpáramo soils are extremely infertile since without
organic matter or fine grains they have practically no ability to hold
exchangeable cations (Pérez, 1992c). Furthermore, in the
superpáramo, the soil surface is recurrently disturbed by needle-ice
activity (a type of ground frost), and soil-moving phenomena such as
frost-heaving and thawing and sorting of material is common
(Pérez, 1987c). In this part of the
high páramo, the mean annual air temperatures are always low (4-6°C),
but the cold does not penetrate very deeply into the soil. Soil temperatures,
at about 30 cm, more or less reflect those of the mean annual temperatures
of the air (Lauer, 1979). Soil temperature does, however, have a
profound effect on nutrient and water availability, root growth, seed survival
and germination, and vegetation zonation (Diemer, 1996; Lauer, 1981;
Pérez, 1987b; A. P. Smith, 1976; Walter & Medina,
1969).
At its middle elevations (i.e., grass
páramo), páramo soils are relatively deep, humic, black or
dark brownish, and acidic with pH ranging from about 3.7 to 5.5.
They are continuously moist or even saturated with water due to the daily
formation of dew or frost and the water-retaining capabilities of the highly
organic, peat-like content.
In the lowermost part of the páramo (i.e., subpáramo),
near Bogotá (Colombia) and elsewhere in the northern Andes, Sturm
(1978) has stated that soils have in common: dark color ("black coloured"),
moderate to high pH and correspondingly low Ca levels, low free P content,
relatively high K and N content and reduced uptake of these elements by
plants, higher than 10% organic content in the top layer, little or no
"podzolic" features, and high water capacity.
For a discussion of the factors that
help to form páramo soils, see Cortés Lombana (1982, 1995).
For soil types in local páramo studies, see Baruch (1979), Botero
(1985), Hofstede (1995c), Pérez (1991c, 1992c, 1996b), Quintanilla
P. (1983b), Rangel Ch. (1989), Salomons (1986), Sánchez M. et al.
(1989), Sevink (1984), Sturm and Rangel Ch. (1985), Thouret and Faivre
(1989), and Vis (1995). For other general references about páramo
soils, see also Del Llano (1990), Jenny (1948), Jenny et al. (1948), Simonson
(1979), Sturm (1994a), Van der Hammen and Ruiz (1984), Van der Hammen and
Santos (1995), Van der Hammen et al. (1983, 1989, In press), Vareschi (1970),
Wright and Bennema (1965), and Zöttle (1970).
Paleohistory and Paleoecology
Reconstruction of the paleoecology of
páramo and high elevation montane forest has been the subject of
study by Thomas Van der Hammen and his associates since the 1960s.
The following is a brief summary of an article by Van der Hammen and Cleef
(1986) that emphasizes the paleohistoric events that gave rise to and further
influenced development of the páramo ecosystem we see today in the
high plain of Bogotá (Colombia). The sequences may be similar
or different in other parts of the northern Andes. Unfortunately,
detailed accounts from other areas are few or not yet available.
The Andes began to arise during the
Paleocene, and during the Miocene they were probably only ridges to low
mountains up to ca. 1000 m elevation. It was not until the beginning
of the Pliocene or slightly later that the northern Andean region uplifted
to its present altitudes. During the Plio-Pleistocene, ca. 4-5 Ma
(million years ago) there was abundant volcanic activity, at which time
elevations above the present treeline came into being. There may
not have been forest yet at the high elevations, since considerable time
was needed for the forest line to have risen from around 2500 m (the level
at that time) up to around 3500 m. The upper Andean forest and páramo
belts evolved more or less simultaneously during the Late Pliocene or Early
Pleistocene (2-4 Ma). There is evidence that an early páramo
vegetation, what Van der Hammen and Cleef call "protopáramo" vegetation,
was present and consisted of Poaceae, Cyperaceae, Asteraceae, Ericaceae,
a Polylepis-Acaena (Rosaceae) type of pollen, and Symplocos
(Symplocaceae), Myrica (Myricaceae), Aragoa (Scrophulariaceae),
Hypericum (Clusiaceae), Miconia (Melastomataceae), Ilex
(Aquifoliaceae), Hydrocotyle (Apiaceae), Borreria (Rubiaceae),
Ludwigia (Onagraceae), Polygonum (Polygonaceae), Valeriana
(Valerianaceae), Plantago (Plantaginaceae), Ranunculus
(Ranunculaceae), Myriophyllum (Haloragaceae), Jamesonia
(Pteridaceae), and Hymenophyllum (Hymenophyllaceae). By 1 Ma
(for the high plain of Bogotá, Colombia, at about 2600 m), there is
evidence for about 15 to 20 repeated alternations of forest and páramo
(i.e., fluctuations of climatic zones) in interglacial and glacial periods.
During this time genera of north temperate origin, such as Alnus
(Betulaceae) ca. 1 Ma and Quercus (Fagaceae) ca. 0.3 Ma, appeared in
the pollen record and must have passed over a Panamanian landbridge.
During the later part of the Quaternary,
ca. 44,000-21,000 yr BP (before present) of the Last Glacial stadial, glacials
and interglacials continued to alternate with some short but severe cold
periods. At that time there were numerous changes in the proportions
between páramo and forest elements, although the páramo flora
became well established and dominated the scene. Between ca. 45,000
yr BP and 25,000 yr BP there was a cold and wet period during which time
glaciation reached its maximum advance. Van der Hammen and Cleef
further explain that during this time the glaciers and forest may even
have been in contact at elevations between 2200 m and 2700 m, and the páramo
belt must have been relatively narrow and wet. On the contrary, between
21,000 yr BP and 14,000 yr BP there was a very cold but dry period during
which time the mean annual temperature may have been 6-7°C lower than
today. Glaciation was not so extensive, but the páramo belt
was broad and dry and covered most of the area above 2000 m (i.e., the
area where present-day forest occurs). This means that páramo
vegetation covered a much greater area than it does today, and that many
of the currently isolated páramos were then united. It also
means that the upper forest line was lowered by 1300-1500 m.
At the beginning of the Holocene (ca.
10,000 yr BP) the climate became much warmer; forest limits rose
to elevations even higher than today and páramo vegetation was restricted
to above 3300-3500 m. The lower elevation forest included Dodonaea
viscosa (Sapindaceae), Myrica (Myricaceae), Myrsine (=Rapanea)
(Myrsinaceae), and Miconia (Melastomataceae); it continued
upwards with Alnus (Betulaceae), and ended at the highest elevations
with Weinmannia (Cunoniaceae) and Quercus (Fagaceae).
During the period from 7500 yr BP to ca. 3000 yr BP temperatures rose about
2°C more, causing another upward shift in the forest line of about
300-400 m higher than today, and thereby reducing the area occupied by
páramo. Finally, at about 2900 yr BP, there was a noticeable
lowering of the temperature that marked the last downward movement of the
forest and páramo belts to their present-day positions. Van
der Hammen and Cleef state that the most important changes in the Holocene
period have been the temperature changes mentioned above, the development
of soils, and the development of peat bogs and soils with increased humidity.
They summarize by saying that the present-day páramo flora and vegetation
is the result of an amalgamation of approximately 4-5 million years of
complicated paleohistoric events.
Some recent publications suggest that
the situation may be more complex or may differ in other parts of the Andes
than that described by Van der Hammen and Cleef (1986). Colinvaux
et al. (1997), for example, refute Van der Hammen's idea that Andean vegetation
zones were compressed and moved in belts during Quaternary times.
Instead they suggest that during times of glacial cooling or Holocene warming
plant associations showed different spatial diversity and were reformed
according to the temperature tolerance of individual species, with heat
intolerant species showing larger displacements.
For additional general references about
geology, glaciation, and paleohistory in the páramo regions of Central
and South America, see Horn (1990b) and Weber (1958) for Costa Rica;
Graham (1973, 1989) and Markgraf (1989) for general Central and South America;
Salgado-Labouriau (1986) and Schubert and Vivas (1993) for Venezuela;
Helmens (1990), Hooghiemstra and Ran (1994), Van der Hammen (1981b, 1989),
and Van der Hammen et al. (1973) for Colombia; and Colinvaux et al.
(1997), Hastenrath (1981), and Wolf (1892) for Ecuador.
Vegetation Zonation
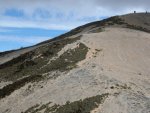 Arenal del Cerro Amarillo |
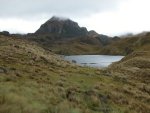 Laguna Patoquinoas |
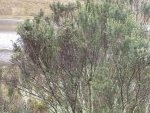 Páramo arbustivo
|
Over the years numerous authors have
given various names to the different vegetation zones and plant associations
within the high Andes. At times the usage of these names can become
confusing and it is difficult to know exactly what is being discussed and/or
compared, but see Huber and Riina (1997) and Jørgensen and Ulloa
U. (1994) for summaries of this nomenclature. When thinking of or
talking about páramo, for example, reference is generally being
made to the open, treeless grasslands with scattered espeletias and shrubs.
Much of the present day páramo vegetation of treeless grasslands,
however, probably has anthropogenic origins, being maintained by cutting,
periodic burning, and grazing; practices mostly intensified within
the last 300 years. Therefore, the natural forest line at which forest
ends and undisturbed páramo begins was probably higher than what
is seen today and above 4000 m elevation in some places. The evidence
for this is that in an undisturbed system, there is usually not an abrupt
end to the forest, not a sharp edge or border, but instead more of a transition
from the tall forest trees to gradually shorter trees with increased elevation,
then small trees and shrubs in a more or less thicket formation, and finally,
above the forest line to the grasses, herbs, and scattered small shrubs
of páramo. As one reaches the natural limits of one zone with
the next, many of the plant species characteristic
of these zones intermix. It must also be remembered that the boundaries
of the vegetation zones and the elevations at which they begin and end
are not fixed. Depending on environmental features, such as topography,
exposure, soils, and general climatic conditions, and human intervention,
timberline may occur anywhere between about 2800 m and 4000(-4800) m in
the northern Andes. Furthermore, on the wetter or windward side of
a mountain or cordillera, the boundary between forest and páramo
(i.e., the contiguous forest line) is higher than on the dry or leeward
side (Lægaard, 1992; Lauer, 1981, 1993; A. P. Smith,
1975b,c, 1994; Troll, 1958, 1959, 1968b, 1973; Verweij, 1995).
This book follows Cuatrecasas (1934,
1954, 1958, 1968) in dividing páramo ideally into three broad zones
based on overall altitude and vegetation structure, with varying degrees
of intergradation. From the highest elevations to the lowest, these
three zones are called superpáramo, páramo (here referred
to as grass páramo), and subpáramo. Superpáramo
may be looked upon as the transition belt or ecotone between the permanent
snow region above and the grass páramo below. Subpáramo
may be seen as the transition belt or ecotone between the grass páramo
above and the montane forest below. A brief summary of these three
zones follows. Other ideas of páramo zonation or modifications
of Cuatrecasas' ideas may be found in papers by Monasterio (1980c) and
Vareschi (1970) for Venezuela, by Cleef (1981b), Espinal and Montenegro
(1963), Fosberg (1944), and Guhl (1982) for Colombia, by Acosta-Solís
(1984), Cañadas Cruz (1983), Harling (1979), Jørgensen and
Ulloa U. (1994), and Ramsay (1992) for Ecuador, by Brack Egg (1986b) for
Peru, or by Cabrera (1957), Fosberg (1967), and Holdridge (1967) for more
general systems of overall vegetation classification that include páramo.
Superpáramo. This
is a narrow zone of vegetation growing on rocky scree and coarse, sandy
soils below the snow-line from about (4000-)4500-4800(-5000) m altitude.
Amongst the three páramo zones it is characterized
by the lowest air temperature, precipitation, soil water-holding capacity,
and nutrient content, and the highest solar radiation and night-frost frequency
(Baruch, 1984). Plants in this zone must be capable of enduring the
daily extreme conditions of coldness and strong radiation, and regular
or frequent snowfalls. Superpáramo is the zone of least disturbance
by man. It is very localized because of its scattered occurrence
only on the highest mountains and has very high endemism. Some superpáramos
occur on mountaintops that are high enough in elevation to have glaciers
in their uppermost regions, while others are without permanent snow.
Vegetation referred to as desert páramo, or sometimes locally called
"arenales," may have so much sand that they look like beaches.
At first glance, superpáramo
often looks from a distance to be bare ground, but is in reality home to
tiny, clumped or scattered plants, such as Azorella pedunculata
(Apiaceae), Hypochaeris sessiliflora, Senecio canescens,
S. nivalis, S. adglacialis, S. supremus, S. comosus,
Pentacalia gelida and Xenophyllum rigidum (=Werneria rigida)
(Asteraceae), Draba pachythyrsa, D. depressa and Eudema nubigena
(Brassicaceae), Arenaria spp. and Cerastium floccosum
(Caryophyllaceae), Pernettya prostrata and Disterigma empetrifolium
(Ericaceae), Astragalus geminiflorus, Lupinus alopecuroides,
L. microphyllus and L. smithianus (Fabaceae), Geranium
multipartitum (Geraniaceae), Luzula racemosa (Juncaceae),
Nototriche jamesonii and N. chimborazoensis (Malvaceae),
Aciachne pulvinata, Agrostis nigritella, Bromus oliganthus,
Poa cucullata, P. trachyphylla, P. orthophylla and Stipa ichu (Poaceae),
Plantago sericea ssp. nubigena (Plantaginaceae), Valeriana
alpifolia (Valerianaceae), Viola pygmaea (Violaceae), the only
páramo gymnosperms Ephedra americana and E. rupestris
(Ephedraceae), and various species of cryptogams including vagrant
ball-forming mosses and unattached lichens.
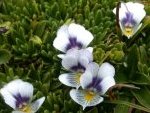 Viola pygmaea |
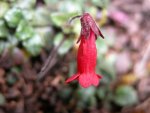 Ourisia chamaedrifolia |
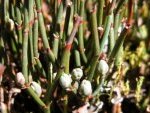 Ephedra americana |
Grass Páramo. [Cuatrecasas
referred to this zone as grass páramo, "páramo propiamente
dicho", or páramo proper.] From about 3500-4100(-4400) m vegetation
of the grass páramo is continuous and plant cover is generally 100%.
It is composed mainly of tussock- or bunch-grasslands dominated by species of
Calamagrostis and/or Festuca. There
is a high proportion of dead shoots among the living that give a yellowish-
or olive-brown to grayish look to the grasslands as a whole (Penland, 1941).
This is the classic area of the genus Espeletia and its
relatives (Asteraceae: Espeletiinae), in local communities called
"frailejonales", which for so many have come to symbolize páramo
vegetation with their columnar, woolly, rosette-plant growth form.
During October and November, in the higher Venezuelan páramos,
there is no greater display of brilliant floral colors anywhere than
when Espeletia schultzii (deep yellow) and Senecio formosus
(violet-maroon) (Asteraceae),
Castilleja fissifolia (bright red, yellow, and green) (Scrophulariaceae),
and Chaetolepis lindeniana (intense magenta) (Melastomataceae) are
in full bloom.
The grass páramo is the most
broadly circumscribed of the three páramo zones. It includes
not only the dominant grassland communities, but also the greatest number
of azonal communities, which are determined by specific factors such as
soil moisture and topography, and páramo growth forms. The
most frequently encountered azonal communities are described below under
the grass páramo, but several are also found to a limited extent
in the superpáramo and subpáramo zones as well. The
grassland communities of the grass páramo zone have suffered greatly
from burning and grazing (see Impact of Burning and Grazing below).
Grass páramo may consist of tall-
and short- grass communities ("pajonales", "pastizales", or "prados") that
include both herbaceous and woody vegetation, but they are dominated by
tussock- or bunchgrasses. In the tall grass communities, with grass up to 1 m
tall, Calamagrostis recta usually dominates on drier sites, while
C. effusa dominates on moister. Short grass communities usually
indicate grazing and burning pressures and are often dominated by species of
Agrostis, Festuca, and Paspalum. The grass Aciachne
pulvinata often forms low or flat cushions with very sharp (to the touch)
leaves in drier-site short-grass páramos. In Colombia, dwarf
bamboos (Chusquea spp.) dominate on slopes with very wet climates in
communities known as bamboo-brakes or "chuscales".
If one takes the time to search between
the bunchgrasses of the open grass páramo, there is an astonishing
array of species of small herbs, e.g., Bomarea spp. (Alstroemeriaceae), Eryngium humile (Apiaceae), Perezia spp. (Asteraceae),
Lysipomia spp. (Campanulaceae-Lobelioideae), Paepalanthus
(Eriocaulaceae), Lupinus spp. (Fabaceae),
Gentiana sedifolia,
Gentianella spp. and Halenia spp. (Gentianaceae), Lachemilla spp. (Rosaceae),
Gunnera magellanica (Haloragaceae), Sisyrinchium spp. and Orthrosanthus chimboracensis (Iridaceae),
Oenothera epilobiifolia (Onagraceae), Ranunculus spp.
(Ranunculaceae), Castilleja fissifolia and Bartsia spp. (Scrophulariaceae), the lycopods Huperzia spp., and the fern genus Jamesonia (Pteridacae). Common
species rooted within the tussock formations include Cerastium spp.
(Caryophyllaceae), Vicia andicola (Fabaceae), Geranium spp.
(Geraniaceae), and Bromus lanatus (Poaceae). Rumex
acetosella (Polygonaceae) is a weedy adventive species that often forms
a red landscape over large disturbed areas, usually after potato or wheat
cultivation. Senecio niveo-aureus (Asteraceae) and related species
in Senecio sect. Culcitium add a stark beauty with
their dense, woolly, whitish to pale yellowish pubescence, which covers
practically the entire plant. Acaulescent rosette-plants in the
genera Oreomyrrhis (Apiaceae), Hypochaeris (Asteraceae),
Acaulimalva (Malvaceae), and Acaena (Rosaceae), and
cushion-plants in genera such as Werneria and Xenophyllum
(Asteraceae), Draba (Brassicaceae), Arenaria (Caryophyllaceae),
and Paepalanthus (Eriocaulaceae) are also common in the grass
páramo. Grass páramo is also rich in small and large
shrubs, such as those genera mentioned in the subpáramo (below),
but they are more scattered in the grass páramo. They include
many species of Baccharis, Diplostephium, Gynoxys,
Loricaria and Pentacalia (Asteraceae),
Hypericum(Clusiaceae), Gaylussacia, Gaultheria, Pernettya and
Vaccinium (Ericaceae), Arcytophyllum (Rubiaceae), and Valeriana (Valerianaceae).
Swampy or boggy azonal sites, called
cushion mires or "turberas", are common, especially in the uppermost grass
páramo (Bosman et al., 1993; Cleef, 1980a. Here species of
the spectacular cushion plant growth form attain their best development,
e.g., Azorella aretioides, A. multifida and A.
pedunculata (Apiaceae), Oreobolus obtusangulus (Cyperaceae),
the moss-like Distichia muscoides (Juncaceae), and Plantago
rigida (Plantaginaceae). The long-lived
cushions often form the substrate for other smaller plants such as
Hypochaeris spp. (Asteraceae), Carex spp. (Cyperaceae),
Disterigma empetrifolium and Pernettya prostrata (Ericaceae),
Gentiana sedifolia and Gentianella spp. (Gentianaceae), Agrostis spp. and Poa
spp. (Poaceae), and Lachemilla spp. (Rosaceae). These cushion "epiphytes"
derive their water and nutrients from the process of litter decomposition
and nurient release taking place within the cushion itself (Sklenár,
1998). Other common species of swampy or boggy sites include Juncus
spp. (Juncaceae), the páramo endemic Castratella piloselliodes
(Melastomataceae), and Valeriana spp. (Valerianacee).
The lichen genera Cladia (Cladoniaceae) and Usnea (Parmeliaceae),
mosses Campylopus (Dicranaceae), Breutelia (Bartramiaceae),
Sphagnum (Sphagnaceae), and liverworts Riccardia (Aneuraceae),
Frullania (Jubulaceae), and Lophozia (Jungermanniaceae) are
also sometimes abundant.
Other wet or flooded azonal communities
such as marshes ("pantanos" or "ciénagas"), seeps, and springs, may
also include bunchgrasses (especially Festuca dolichophylla),
but with more species of sedges (Carex, Eleocharis, etc.) and mosses
such as Drepanocladus (Amblystegiaceae) and Sphagnum (Sphagnaceae).
Also found are the various species of Lilaeopsis and Hydrocotyle
(Apiaceae), Oritrophium peruvianum (Asteraceae), Plagiobothrys
linifolius
(Boraginaceae), Draba lindenii (Brassicaceae), Stellaria media
(Caryophyllaceae),
Halenia spp. (Gentianaceae), Myriophyllum
quitense (Haloragaceae), Juncus spp. (Juncaceae),
Huperzia spp. (Lycopodiacee), Rumex tolimensis (Polygonaceae)
to 4-5 m tall, Caltha sagittata and Ranunculus praemorsus (Ranunculaceae),
and Mimulus glabratus and Pedicularis incurva (Scrophulariaceae).
Shallow pool, lake, and river communities (Figs. 15C-D) include Callitriche
spp. (Callitrichaceae), Elatine spp. (Elatinaceae), Myriophyllum
spp. (Haloragaceae), Isoëtes spp. (Isoetaceae), Cortaderia
spp. (Poaceae), Potamogeton spp. (Potamogetonaceae), and Ranunculus
spp.
Rock ledge and cliff communities harbor
another distinct group of interesting plants including Draba spp.
(Brassicaceae), Luzula racemosa (Cyperaceae), Escallonia
myrtilloides and Ribes hirtum (Grossulariaceae),
Calceolaria spp. (Scrophulariaceae) and numerous fern species in the
genera Asplenium and Cystopteris (Aspleniaceae) and
Elaphoglossum and Woodsia (Dryopteridaceae).
Trees of the genus Polylepis
(Rosaceae), with their characteristic reddish, exfoliating bark and strangely
contorted trunks and branches, may form localized, isolated woodlands or
forest communities to over 4000 m elevation within otherwise grass
páramo. They are often found on scree slopes, near the shelter
of rock cliffs, or in river valleys. It is interesting that within the
Polylepis forest there is a noticeable
drop in plant diversity compared to the surrounding grass páramo
(páramo species are probably shade intolerant), and furthermore
that certain plants grow only under Polylepis. Polylepis forest
is still relatively underexplored and an overall study of its flora would
be very interesting (but see Arnal, 1983; Fjeldså and Kessler,
1996; and Hueck, 1960a).
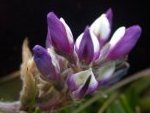 Lupinus |
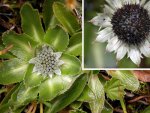 Eryngium humile |
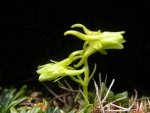 Halenia spp. |
Subpáramo. The lowest
zone, called subpáramo, is also the most diverse and floristically
showy. It is a shrub-dominated transition zone at (2800-)3000-3500
m elevation made up of elements from forest below and the grass
páramo above. It is often a mosaic of shrubs and small scattered
trees, gradually reduced in size, giving way to scrub and low vegetation
of dwarf shrubs, grasses, and herbs of the grass páramo above.
Sometimes local changes in topography and soils may give way
to different microclimatic conditions and so small patches of forest may
be present. Where the ecotone between forest and páramo is
abrupt, or where isolated shrub-tree islands are found within páramo,
it is usually brought about by anthropogenic means, especially cutting,
burning, and grazing. Therefore, it is possible that
subpáramo consists of nearly all secondary communities.
The subpáramo communities, often
known as scrub, thickets, "chaparrales" or "mattorales", are composed
predominantly of shrubby or woody vegetation that is sometimes lacking
or rare in the Andean forest below. These include species of the
genera Ilex (Aquifoliaceae), Ageratina, Baccharis, Chuquiraga,
Diplostephium, Gynoxys, Loricaria, Senecio
and Stevia (Asteraceae), Berberis (Berberidaceae),
Siphocampylus (Campanulaceae-Lobelioideae), Hypericum (Clusiaceae),
Coriaria (Coriariaceae), Desfontainia (Desfontainiaceae),
Bejaria, Cavendishia, Gaultheria, Macleania, Pernettya, Semiramisia,
Themistoclesia and Vaccinium (Ericaceae), Brachyotum, Chaetolepis, Miconia and Monochaetum (Melastomataceae),
Myrsine (Myrsinaceae), Monnina (Polygalaceae), Rubus (Rosaceae),
Arcytophyllum (Rubiaceae), Aragoa and Calceolaria
(Scrophulariaceae), Symplocos (Symplocaceae), and Ternstroemia
(Theaceae). Woody epiphytes in the Ericaceae
(e.g., Disterigma, Plutarchia, Sphyrospermum, Thibaudia) and
Loranthaceae (e.g., Aetanthus) (Figs. 10D and 14B) are also
common.
Genera forming low forests of small
trees up to eight meters tall, often in tree islands known as "bosques
achaparrados" include: Oreopanax and Schefflera (Araliaceae),
Gynoxys, Diplostephium and Senecio (Asteraceae), Buddleja
(Buddlejaceae), Weinmannia (Cunoniaceae), Bejaria (Ericaceae),
Escallonia (Grossulariaceae), Miconia (Melastomataceae),
Myrsine (Myrsinaceae), and Hesperomeles (Rosaceae).
Subpáramo has many common names,
of which the most frequently used are: páramo forest, páramo
thicket, shrubby páramo, subpáramo woodland, subpáramo
chaparral, subpáramo elfin forest, and tropical subalpine forest
(in English); paramillo, paramito, bosquecillo de páramo,
matorral de páramo, matorral de subpáramo, bosque paramero,
bosque subparamero, bosque enano, bosque musgoso de subpáramo, bosquete
andino, and chirivital (in Venezuela) (in Spanish); and paramillo
thicket and paramillo scrub (mixed Spanish and English).
In general terms, subpáramo is
the most difficult of the three páramo zones to define, because
it has been greatly extended and expanded both horizontally and vertically
(downwards) by human disturbance and habitat destruction over hundreds,
or perhaps thousands of years. This is especially due to cutting
and burning at the upper forest line for herding and agricultural purposes.
Correspondingly, forest lines appear to have been lowered by as much as
several hundred meters by human interference (Lægaard, 1992), and
many species that were more restricted to relatively high elevations appear
to have colonized lower life zones (Budowski, 1968). Much of both
subpáramo (non-controversial) and grass páramo (somewhat
controversial) now occur in areas that were probably covered with upper
montane forests in the past (Lægaard, 1992).
Detailed classifications of páramo
vegetation have been constructed utilizing relevés in the Zürich-Montpellier
approach and described following the international "Code of Phytosociological
Nomenclature" (Barkman et al., 1976). This system divides vegetation
into units or communities on the basis of floristic, physiognomic, and
ecologic similarities, and usually employs a detailed classification of
the vegetation consisting of a syntaxonomical hierarchy (subassociation,
association, alliance, order, class, etc.). For further explanation
of this methodology, see R. Becking (1957), Braun-Blanquet (1964), Mueller-Dombois
and Ellenberg (1974), and Westhoff and Van der Maarel (1973). For
applied examples of this methodology, with lists of species characterizing
the various plant communities, see Aguirre and Rangel Ch. (1976), Cleef
(1981b, including figs. 11-79), Franco R. et al. (1986), Lozano C. and
Schnetter (1976), Sturm and Rangel Ch. (1985, including figs. 4-7), and
Vargas Ríos and Zuluaga (1980). Páramo studies using
other quantitative methods may be seen in Baruch (1984) and Fariñas
and Monasterio (1980) for Venezuela, and in Balslev and de Vries (1982),
Grubb et al. (Unpublished), Muñoz et al. (1985), and Ramsay (1992)
for Ecuador.
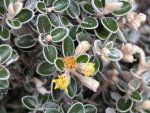 Gynoxys miniphylla |
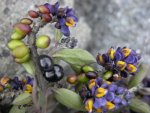 Monnina crassifolia |
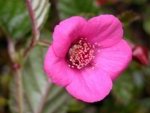 Rubus coriaceus |
Morphological and Physiological Adaptations
Since páramo is a high elevation
tropical ecosystem, certain characteristic physical, chemical, and climatic
features affect the biological functioning of the organisms that live there.
Therefore, the plants that grow in these areas must be adapted to:
1) high elevation air (with less water content and lower partial pressures
of gasses such as O2 and CO2), 2) low temperatures (in the shade
the annual mean temperature decreases about 0.6°C for each 100 meters
increase in elevation, whereas in full sunlight, it increases with elevation
because the atmosphere neither absorbs nor disperses as much radiation
energy as in the lower regions), 3) intense ultra-violet radiation
(highest in equatorial high mountains, but controlled by frequent fog and
cloud cover), 4) rapid changes in insolation resulting in quick absorption
or loss of heat, 5) drying effect of winds (by increasing transpiration
to the point of desiccation), 6) physiological dryness (due to the combination
of low temperatures, intense transpiration during sunny periods, and drying
effects of winds, along with high soil acidity and high osmotic pressure
of soil water making root water-absorption difficult), and 7) physical
damage from hail and possibly snow (Acosta-Solís, 1984; Cleef,
1981b; Cuatrecasas, 1968; Little, 1981; Mani, 1980;
G. Sarmiento, 1986; A. P. Smith, 1981; Vareschi, 1970;
Young, pers. comm.). Consequently, growth and decomposition are slow,
primary productivity is low, and natural succession of the vegetation takes
a long time, especially when woody species are involved (Ferweda, 1987;
Hofstede, 1995c; Horn, 1989, 1997; Janzen, 1973; Ramsay
& Oxley, 1996; Salamanca V., 1991; L. Sarmiento M. et al.,
1990; A. P. Smith, 1981; Sturm, 1978; Williamson et al.,
1986). It is important to remember that there is no strong temperature
seasonality, no marked change from summer to winter as in the temperate
regions, but that in páramo growth is continuous throughout the
year and that great changes in temperature (and to a certain extent precipitation)
occur every day (diurnally). Smith and Young (1987) noted that "Many
aspects of morphology and physiology seem to provide escape from, or tolerance
to, extreme diurnal climatic fluctuations."
Recent studies have shown how giant
Andean rosettes (e.g., Espeletia and Puya), have evolved adaptations that
favor temperature insulation and the maintenance of a positive water balance
under the severe conditions of the páramo environment. Adaptations
to low temperatures include freezing avoidance mechanisms such as supercooling
of adult leaves (Goldstein, Rada & Azócar, 1985; Larcher,
1975; Rada R. et al. 1985a); insulation by retention of dead
leaves (marcescent leaves), which protects rosette stems from freezing
(Goldstein & Meinzer, 1983; Rada R. et al., 1985a; A. P.
Smith, 1979); parabolic leaf geometry and nyctinastic movements of
the leaves, which result in protection of the apical leaf bud from freezing
(O. Hedberg, 1964; Larcher, 1975; A. P. Smith, 1974b);
thermal buffering by mucilaginous fluids secreted by the leaf bases, which
protect apical buds (Smith & Young, 1987); dense leaf pubescence,
which reduces transpiration (Baruch, 1972) and increases leaf temperature
(Baruch, 1975; Meinzer & Goldstein, 1985; Meinzer, Goldstein
& Rada, 1994; Miller, 1986, 1994); tall aerial stems, which
protect buds against the low minimum nighttime temperatures at ground level
(Meinzer, Goldstein & Rada, 1994; A. P. Smith, 1980); and
contractile roots in juvenile plants, which draw the developing stem into
the ground (Smith in Smith & Young 1987).
Rosette adaptations to low moisture
levels include changes in the method of CO2 assimilation, such as a switch
to the CAM photosynthetic pathway (Baruch & Smith, 1979; Medina,
1974); and a well-developed water-storing pith tissue, which can
be used particularly during early morning hours when cold or frozen soils
limit water uptake during that period of high transpiration (Goldstein
& Meinzer, 1983; Goldstein et al. 1984; Meinzer & Goldstein,
1986; Meinzer, Goldstein & Rundel, 1994; Meinzer et al.
1985). Some rosette plants, like Draba chionophila (Brassicaceae),
which grows up to ca. 4800 m in the Venezuelan Andes, are freeze-tolerant
(i.e., freezing injury occurs only when temperatures drop below the temperature
at which extracellular ice formation begins) (Azócar et al., 1988;
Goldstein et al., 1994; Pfitsch, 1994). For reviews of general
adaptive radiation in Espeletia and other plants of the high Andes,
see also Beck (1994), Goldstein et al. (1994), Hedberg and Hedberg (1979),
Monasterio (1986b), Monasterio and Sarmiento (1991), Ramsay (1992), Rundel
et al. (1994), and Smith and Young (1987).
Some of the physiological and morphological
adaptations discussed above work in combination, that is, not only as avoidance/tolerance
mechanisms against a cold environment, but also as useful adaptations against
a hot environment resulting from frequent fires. Adaptations
to high elevation by páramo plants result in the characteristic
growth forms discussed below.
Growth Forms
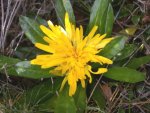 Hypochaeris |
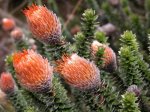 Chuquiraga |
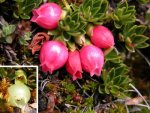 Disterigma |
Most high elevation tropical plant communities
have a characteristic physiognomy, which repeats in geographically disjunct
areas of the world where they occur, for example, South America, East Africa,
and Hawaii (Cuatrecasas, 1968; O. Hedberg, 1964, 1992; Hedberg
& Hedberg, 1979; Raunkiaer, 1934; Troll, 1958; Vareschi,
1970). The growth forms (sensu lato) that characterize this physiognomy
are examples of convergent evolution, the forms having evolved independently
in several different plant families on distant continents in response to
the unique high altitude tropical environments. These growth forms
often result from the ecological and morphological adaptations mentioned
above. Many are also apparantly adapted to survive fire (Lægaard,
1992; Young & León, 1991).
While the tree is the dominant growth
form in the forest, it is essentially absent from páramo (except
the genus Polylepis and a few associated species). Apparently
it cannot survive at such altitudes. In fact, tree growth ceases
when soil tempertures drop to 6-10°C (Larcher, 1975; Lauer, 1979a,
1981; Walter & Medina, 1969a,b). Páramo, however,
has its own important and conspicuous growth forms (not all of which are
strictly found in páramo), such as bunchgrasses, rosettes plants,
cushion plants, microphyllous and dwarf shrubs, vagrant plants, and geophytes.
The presence of rosette plants (some of giant size), for example, is probably
a good general indicator of páramo and seems to be one of the clearest
distinctions between high elevation areas of tropical and temperate latitudes
(A. P. Smith, 1994). The most characteristic growth forms of the
high elevation páramos are summarized below. See also Balslev
and de Vries (1991), O. Hedberg (1964, for comparisons with Afroalpine
plants), Hedberg and Hedberg (1979), and Ramsay and Oxley (1997).
Rosette Plants. This growth
form gives páramo vegetation its distinctive character. Two kinds
of rosette plants have been described:
Stem rosette.-- The most typical
and well known growth form of páramo is the columnar woolly rosette
plant. Members of the genus Espeletia (Asteraceae), the so-called
"frailejón" (or literally translated "big friar") because of the
grayish woolly coat of pubescence, are the classical example. These
plants produce an erect, normally unbranched, thick-woody stem tightly
encased by the dense bases of old leaves (Cuatrecasas, 1968). The
erect stems may be as tall as 15 meters in undisturbed páramo.
Lateral inflorescences are produced from the single aerial meristem.
Other examples of this growth form are the fern Blechnum schomburkii
(Blechnaceae) and Plantago sericea ssp. perrymondii (Plantaginaceae).
It has been shown that woolliness is a response to ultra-violet light and
is associated with thermoregulation, the retarding of evaporation, and
general protection from UV light (Miller, 1994).
Acaulescent rosette.-- These plants
develop thick, perennial, tap-roots and a dense rosette of leaves at the
ground level. The flowering stems may be very short with the flowers
hidden in and amongst the leaf bases, or longer thereby lifting the flowers
above the ground surface. Some plants, such as Puya spp. (Bromeliaceae),
produce giant, bulky inflorescences several meters tall. In all cases,
the buds that form the flowers originate in the axils of
the rosette leaves, which are sunken a few centimeters below the ground.
Acaulescent plants thereby protect their buds from fire and frost.
Experiments have shown that the mean temperature within the rosette is
higher (by about 6°C) than in the surrounding air (Hedberg & Hedberg,
1979), and that these plants seem to buffer temperature variations to such
an extent that they are able to avoid both positive and negative extremes
(Goebel, 1891; O. Hedberg, 1964). Examples are shown by the
genera Hypochaeris and Werneria (Asteraceae),
Lysipomia (Campanulaceae-Lobelioideae), Paepalanthus (Eriocaulaceae),
Lupinus alopecuroides (Fabaceae), Acaulimalva
and Nototriche (Malvaceae), Rumex tolimensis (Polygonaceae),
Ranunculus gusmanni (Ranunculaceae), Acaena cylindristachya
(Rosaceae), Valeriana plantaginea (Valerianaceae), and Viola
(Violaceae).
Cushion Plants. These plants
form a flat, convex, or hemispherical cushion as the result of the regular
outward branching of dense radially oriented buds. Each
branch has a small rosette of leaves at the tip and only the outer and
upper leaves are green and living; the interior of the cushion consists
of a peaty mass, the remains of dried leaves, accumulated humus, dust,
soil, and rain water, all of which protect the buds and stems from wind,
desiccation and predation, and provide a reservoir of water and nutrients
(Sklenár, 1998). Like in rosette plants, the mean temperature
is higher within the cushion than at the cushion surface, thereby protecting
the buds from cold temperatures. The plants are often very prickly
and hard to the touch, yet firm enough that one is able to walk on top
of certain species, such as in Azorella (Apiaceae), Plantago
(Plantaginaceae) (Figs. 9C and 19), and Distichia (Juncaceae).
Many different species, in different families, form cushions
of different sizes, from flat or only a few centimeters tall to cushions
over one meter tall and several meters in diameter, for example Azorella
pedunculata. In moist or humid sites, where cushions are more
frequently found, Distichia muscoides and Plantago rigida are dominant species, while in drier
places Azorella pedunculata and A. aretioides are common.
Other genera that produce cushions include Werneria pygmaea and
Xenophyllum spp. (Asteraceae), Draba aretioides
(Brassicaceae), Arenaria spp. (Caryophyllaceae), Oreobolus
spp. (Cyperaceae), Disterigma empetrifolium and Pernettya
prostrata (Ericaceae), Paepalanthus lodiculoides (Eriocaulaceae),
Geranium spp. (Geraniaceae), Aciachne pulvinata (Poaceae),
Calandrinia acaulis (Portulacaceae), Valeriana rigida
(Valerianaceae), and Xyris subulata (Xyridaceae). See O. Hedberg
(1964, 1992), Heilborn (1925), Sklená_ (1998), and Rauh (1939) for
extensive discussions of morphology and adaptation of cushion plants to
high elevation environments.
Bunchgrasses (or Tussock Grasses).
This growth form is the most widespread in the páramo. In
undisturbed areas, grasses may average 1-1.5 m tall with a coverage of
up to 100%. Members of the grass and sedge families frequently form
tufts or dense bunches of stems (culms) with rigid, pointed, tubular or
inrolled leaves. These dense tufts in which the dead leaves are
maintained and decay on the plant, along with the culms, provide good insulation
for the buds and young leaves from cold temperatures, high radiation, evaporation,
and high heat of fires to 500°C (Ramsay, 1992; Ramsay & Oxley,
1996). Much of their regeneration takes place through the production
of vegetative buds near the ground. Here the tufts are very dense
and living shoots are found along with dead culms and leaves. These
tufts protect the vegetative buds. The most common species are Calamagrostis
recta and C. effusa (Poaceae); other important genera and species are
Carex and Uncinia (Cyperaceae), Cortaderia spp., Festuca
dolichophylla, F. tolucensis, Stipa spp., and Lorenzochloa
erectifolia (Poaceae). See Hofstede (1995c), Ramsay (1992), and
Ramsay and Oxley (1997) for additional discussion of how the bunchgrass
growth form is an adaptation to fire in the páramo environment.
Microphyllous Shrubs. These
shrubs are characterized by dense foliage of small, xeromorphic leaves,
sometimes with many of the following combinations of adaptations in the
same species, all acting as protection from ultraviolet light and/or the
reduction of transpiration (O. Hedberg, 1964; Larcher, 1975).
Examples of genera and/or species with hard or sclerophyllous leaves include
Gaultheria anastomosans and Gaylussacia buxifolia (Ericaceae),
and Miconia summa (Melastomataceae); squamous or rolled leaves
Baccharis revoluta and Diplostephium revolutum (Asteraceae),
and Miconia salicifolia (Melastomataceae); imbricate leaves
Loricaria
(Asteraceae) and Aragoa cupressina (Scrophulariaceae);
aciculate or spine-tipped leaves Chuquiraga (Asteraceae), Hypericum laricifolium (Clusiaceae),
and Valeriana microphylla (Valerianaceae); and densely tomentose-pubescent
leaves Diplostephium eriophorum and Pentacalia guicanensis (Asteraceae),
and Gaultheria lanigera (Ericaceae).
The so-called bouquet plants ("plantas
en ramilletes de florones") are a subset of microphyllous shrubs with a
special habit. These plants feature an increase in the size of an
individual flower in relation to the total appearance of the plant (e.g.,
Bidens humilis), or the dense aggregation of many small flowers
into a bouquet of dense flowering stalks (Vareschi, 1970). This is
considered an adaptation to make the flowers more attractive to pollinators
by forming a large and noticeable splash of color that attracts insects
from a distance, as in species of Draba (Brassicaceae), Gentianella (Gentianaceae), and Chaetolepis (Melastomataceae).
Prostrate Dwarf Shrubs.
These are small, woody plants that rarely produce shoots over 0.75 m tall.
The special feature separating them from microphyllous shrubs is that they
have a larger part of their branch system protected below or upon the soil
surface. They are often prostrate, growing laterally along the ground.
Sometimes entire branching systems occur underground and only the current
year's growth is seen above ground. This growth form often has its
regenerative buds below ground where they are protected from fire and frost.
Examples are found in Bidens and Senecio (s.l.) (Asteraceae),
Lupinus and Astragalus (Fabaceae), Pernettya and
Disterigma (Ericaceae), and Arcytophyllum (Rubiaceae).
Geophytes. These are herbs
that survive the unfavorable periods of the year (including times of fire)
by means of subterranean organs, such as succulent roots, rhizomes, stolons,
tubercules, or bulbs (Lægaard, 1992; Raunkiaer, 1934;
Vareschi, 1970). Examples of geophytes include Orthrosanthus chimboracensis
(Iridaceae), the genus Stenomesson (Amaryllidaceae),
Altensteinia and Gomphychis (Orchidaceae), and the fern Ophioglossum
crotalophoroides (Ophioglossaceae).
Vagrant Plants. These plants
grow free, unattached to the substrate, and are found in many biomes throughout
the world (Pérez, 1994a, 1997b). They are found in the superpáramo
zone, where frost-heaving is a common phenomenon, and only in cryptogamic
plants such as the fruticose lichen Thamnolia vermicularis (family
uncertain) and an acrocarpous moss Grimmia longirostris (Grimmiaceae).
These plants have been variously referred to as erratic, vagant, vagrant,
solifluction floaters, errant cryptogams, and sometimes globular mosses
or moss balls when their growth shape becomes more spherical (see Pérez,
1997b for more details). Cryptogams as a whole (i.e., bryophytes
and lichens) have been considered a true growth form by Cuatrecasas (1968),
Griffin (1979), and Ramsay (1992), or as composed of several growth forms
by Ramsay and Oxley (1997).
Flora
On a geological timescale the páramo
flora is young, the so-called protopáramo vegetation of Van der
Hammen and Cleef (1986) having evolved during the Late Pliocene or Early
Pleistocene, some 2-4 Ma. Páramo environments suitable for
plant colonization on an extensive scale, however, have only been available
since the Quaternary (Simpson, 1975; Van der Hammen, 1974;
Van der Hammen & Cleef, 1986). The youth of the páramo
flora is also evidenced by the presence of relatively few endemic or near
endemic genera (23 genera, ca. 5% based on this work) and the absence of
any endemic families in the vascular flora. Although the páramo
ecosystem occupies no more than 2% of the land area of the countries in
which it is found, the flora is extremely diverse. In fact, the páramo
flora is the richest high mountain flora of the world (Smith & Cleef,
1988). The páramo flora has evolved in various ways:
by adaptation of lower elevation plants (i.e., tropical elements) to high
elevation environments, by immigration (i.e., dispersal) of cool-adapted
plants from north and south temperate regions, and by speciation through
isolation from within (i.e., autochthonous element). For discussion
of these and other ideas, see Chapman (1917), Chardon (1938), Simpson (1975),
Simpson and Todzia (1990), Van der Hammen (1972a,b), and F. Vuilleumier
(1970).
Table I. Numbers of
families, genera, and species of major plant groups in the páramo. |
|
Taxonomic Group |
No. Families |
No. Genera |
No. Species |
Non-Vascular Plants |
130 |
365 |
1298 |
Lichens* |
45 |
114 |
465 |
Mosses |
51 |
163 |
544 |
Hepatics |
34 |
88 |
291 |
Vascular Plants |
124 |
500 |
3399 |
Ferns/Fern Allies |
22 |
52 |
352 |
Gymnosperms |
1 |
1 |
2 |
Angiosperms |
101 |
447 |
3045 |
Monocots |
16 |
101 |
634 |
Dicots |
85 |
346 |
2411 |
TOTAL |
254 |
865 |
4697 |
*Lichenicolous fungi are not included in this table. |
|
General Floristic Diversity.
For the non-vascular plants, the lists herein presented include 114 genera
in 45 families of lichens (excluding lichenicolous fungi), 163 genera in
51 families of mosses, and 88 genera in 34 families of hepatics, for a
total of 365 genera and 1298 species (see
Table I). For the vascular plants, the lists include 52 genera in
22 families of ferns and fern allies, 1 genus in 1 family of gymnosperms, 101
genera in 16 families of monocotyledons, and 346 genera in 85 families of
dicotyledons, for a total of 500 genera and 3399 species (see
Table I). Tables II-VI show
the largest families and genera of páramo lichens, mosses, hepatics,
ferns and fern allies, and flowering plants, respectively.
Table VII lists the genera endemic to
páramo and notes those that are monotypic. Luteyn (1992)
provided a preliminary estimate of specific endemism of páramo vascular
plants as high as 60%. However, it is now realized that species numbers,
limits, and distribution patterns are too poorly known and that a realistic
approximation of species endemism in the páramo is not yet possible.
As a result, detailed estimates of overall specific endemism and the geographical
origins and relationships of the páramo flora have not been calculated
from the data herein presented.
Non-Vascular Plants. --
In this study, 1298 species of non-vascular plants have been found throughout
the geographical and elevational range of páramo as here defined
(Table 1), of which 36% are lichens
(lichenicolous fungi are not included), 42% mosses, and 22% hepatics.
Within the lichens,
Table II shows the
10 largest families and genera of páramo lichenized fungi.
At the family level, Parmeliaceae (25 genera and 159 species) are by far
the most diverse, with over three times as many genera and species as the
next closest families Physciaceae (8 gen.) and Cladoniaceae (45 spp.),
respectively. Four of the ten most speciose páramo genera
are also Parmeliaceae -- Hypotrachyna (50 spp.), Oropogon
(21 spp.), Xanthoparmelia (18 spp.), and Parmotrema (13 spp.)
-- accounting for just over 100 species. The second most speciose
family is Cladoniaceae, with Cladonia having 38 species. Additional
general comments about lichens are given by Ahti (1992) and Sipman (1992,
and below in the introduction to his checklist).
Table III shows the 10 largest families and genera of páramo
mosses. At the family level, Dicranaceae (17 gen. and 67 spp.), Bryaceae
(10 gen. and 65 spp.), and Pottiaceae (19 gen. and 63 spp.) are the most
diverse. The most speciose genera are Campylopus (Dicranaceae,
37 spp.), Sphagnum (Sphagnaceae, 27 spp.), and Zygodon
(Orthotrichaceae, 21 spp.). Additional general comments about mosses
are given below by Churchill and Griffin in the introduction to their
checklist. Table IV shows
the 10 largest families and genera of páramo hepatics.
Lejeuneaceae (16 gen. and 38 spp.) and Jungermanniaceae (11 gen. and 31
spp.) are the most diverse families in terms of both genera and species.
The most speciose genera are Riccardia (Aneuraceae, 20 spp.),
Metzgeria (Metzgeriaceae, 20 spp.), Plagiochila
(Plagiochilaceae, 18 spp.), Frullania (Jubulaceae, 13 spp.), and
Bazzania (Lepidoziaceae, 13 spp.). Additional general comments
about liverworts are given below by Gradstein in the introduction to his
checklist.
Table II Ten largest
families and genera of páramo lichenized fungi* (prepared by
H. Sipman). |
|
Family (no. gen./spp.) |
Genus (and family) (no. spp.) |
Parmeliaceae (25/159) |
Hypotrachyna (Parmeliaceae) (50) |
Cladoniaceae (3/45) |
Cladonia (Cladoniaceae) (38) |
Physciaceae(8/27) |
Leptogium (Collemataceae) (25) |
Collemataceae (2/26) |
Oropogon (Parmeliaceae) (21) |
Lobariaceae (3/24) |
Stereocaulon (Stereocaulaceae) (19) |
Stereocaulaceae (1/19) |
Xanthoparmelia (Parmeliaceae) (18) |
Peltigeraceae (3/13) |
Heterodermia (Physciaceae) (17) |
Ramalinaceae (1/12) |
Sticta (Lobariaceae) (13) |
Lecanoraceae (4/11) |
Parmotrema (Parmeliaceae) (13) |
Pannariaceae (4/10) |
Ramalina (Ramalinaceae) (12) |
*Lichenicolous fungi are not included in this table.
|
|
Table III.
Ten largest families and genera of páramo mosses (prepared by S.
P. Churchill and D. Griffin III). |
|
Family (no. gen./spp.) |
Genus (and family) (no. spp.) |
Dicranaceae (17/67) |
Campylopus (Dicranaceae) (37) |
Bryaceae (10/65) |
Sphagnum (Sphagnaceae) (27) |
Pottiaceae (19/63) |
Zygodon (Orthotrichaceae) (21) |
Bartramiaceae (7/40) |
Bryum (Bryaceae) (18) |
Orthotrichaceae (3/36) |
Leptodontium (Pottiaceae) (16) |
Sphagnaceae (1/27) |
Orthotrichum (Orthotrichaceae) (14) |
Amblystegiaceae (9/19) |
Breutelia (Bartramiaceae) (13) |
Brachytheciaceae (7/18) |
Daltonia (Daltoniaceae) (13) |
Polytrichaceae (6/16) |
Macromitrium (Macromitriaceae) (13) |
Grimmiaceae (4/17) |
Schizymenium (Bryaceae) (11) |
|
Table IV. Ten largest families and
genera of páramo hepatics (prepared by S. R. Gradstein). |
|
Family (no. gen./spp.) |
Genus (and family) (no. spp.) |
Lejeuneaceae (16/38) |
Riccardia (Aneuraceae) (20) |
Jungermanniaceae (11/31) |
Metzgeria (Metzgeriaceae) (20) |
Lepidoziaceae (6/29) |
Plagiochila (Plagiochilaceae) (18) |
Aneuraceae (2/21) |
Frullania (Jubulaceae) (13) |
Metzgeriaceae (1/20) |
Bazzania (Lepidoziaceae) (13) |
Plagiochilaceae (2/19) |
Anastrophyllum (Jungermanniaceae) (8) |
Geocalycaceae (7/18) |
Lepidozia (Lepidoziaceae) (8) |
Gymnomitriaceae (5/14) |
Leptoscyphus (Geocalycaceae) (7) |
Jubulaceae (1/13) |
Isotachis (Balantiopsidaceae) (6) |
Balantiopsaceae (2/7) |
Cephaloziella (Cephaloziellaceae) (6) |
|
Marsupella (Gymnomitriaceae) (6) |
|
Radula (Radulaceae) (6) |
|
Vascular Plants. -- In this study,
3399 species of vascular plants have been found from throughout the geographical
and elevational range of páramo
(Table 1), of which 10.4% are ferns
and fern allies, 0.06% gymnosperms, and 89.6% angiosperms. Of the
angiosperms (flowering plants), 21% are monocots and 79% are dicots.
Table V
shows the largest families and genera within the páramo ferns and
fern allies. Dryopteridaceae, Lycopodiaceae, Polypodiaceae, and
Pteridaceae are the largest and most diverse families at the generic
and specific levels. At the generic level, Polypodiaceae (14 gen.)
are the most diverse, with twice as many genera as the next closest
family Pteridaceae (7 gen.). At the specific level, Dryopteridaceae
are the largest due to the numerous species of Elaphoglossum
(65 spp.), this genus is also the largest of the páramo pteridophytes.
Huperzia (Lycopodiaceae) with 60 spp. is the second largest genus
(or the largest with 69 if considered as Lycopodium in the broad
sense), followed by Hymenophyllum (20 spp.), Isoëtes
and Jamesonia (18 spp. each).
Table VI shows the composition of the páramo flowering plants
in terms of the 15 largest families and genera. In this study, the
Asteraceae are the largest family by far in both numbers of genera and
species. The data from this study show that Asteraceae are two and
a half times larger than the Poaceae in numbers of genera (101 gen. vs.
41 gen.) and nearly four times larger in numbers of species (858 spp. vs.
227 spp.). Four of the five most speciose páramo genera are
Asteraceae -- Pentacalia (89 spp.), Senecio
s.s. (69 spp.), Diplostephium (70 spp.), and Espeletia s.s.
(61 spp.) -- accounting for nearly 300 species. Asteraceae also have
the highest number of endemic genera of any páramo vascular plant
family (16 genera, or 70% of the endemic páramo genera). The
largest genera of Poaceae are Festuca (38 spp.), Calamagrostis
(36 spp.), Agrostis (24 spp.), and Poa (20 spp.). The
Orchidaceae are surprisingly diverse in the páramo with 25 genera
and 152 species herein recorded, although (as discussed below) their tabulation
has proven difficult and their numbers may be questioned. It is interesting
to note that in virtually all páramo studies that have been published,
the Asteraceae are always the largest family in numbers of species and
genera, followed closely by Poaceae. Other families (in alphabetical
order) that rank consistently high in overall importance in the páramo
ecosystem include Apiaceae, Brassicaceae, Bromeliaceae, Cyperaceae, Ericaceae,
Gentianaceae, Melastomataceae, Orchidaceae, Rosaceae, and Scrophulariaceae.
There is also a trend in the upper páramo (i.e., superpáramo
at ca. 4000+ m) for certain families, often with a more north-temperate
element, to become increasingly important. For example, the Apiaceae,
Brassicaceae, Caryophyllaceae, Fabaceae, Gentianaceae, Malvaceae, Poaceae,
and Valerianaceae become more conspicuous, while the Bromeliaceae, Melastomataceae,
Orchidaceae, Rubiaceae, and Solanaceae decline (see also table 9 in Jørgensen
& Ulloa U., 1994).
Table V.
Ten largest families and genera of páramo ferns and fern allies. |
|
Family (no. gen./spp.) |
Genus (and family) (no. spp.) |
Dryopteridaceae (5/77) |
Elaphoglossum (Dryopteridaceae) (65) |
Lycopodiaceae (3/69) |
Huperzia s.s. (Lycopodiaceae) (60) |
Polypodiaceae (14/60) |
(Lycopodium s.l. =69) |
Pteridaceae (7/43) |
Hymenophyllum (Hymenophyllaceae) (20) |
Hymenophyllaceae (1/20) |
Isoëtes (Isoetaceae) (18) |
Isoetaceae (1/18) |
Jamesonia (Pteridaceae) (18) |
Thelypteridaceae (1/13) |
Eriosorus (Pteridaceae) (14) |
Aspleniaceae (2/11) |
Thelypteris (Thelypteridaceae) (13) |
Blechnaceae (1/9) |
Polypodium (Polypodiaceae) (12) |
Cyatheaceae (1/6) |
Melpomene (Polypodiaceae) (11) |
|
Asplenium (Aspleniaceae) (10) |
|
Table VI. Fifteen largest
families and genera of páramo flowering plants. |
|
Family (no. gen./spp.) |
Genus (and family) (no. spp.) |
Asteraceae (101/858) |
Pentacalia (Asteraceae) (89) |
Poaceae (41/227) |
Senecio s.s. (Asteraceae) (69) |
Orchidaceae (25/152) |
(Senecio s.l. =172) |
Scrophulariaceae (14/144) |
Diplostephium (Asteraceae) (70) |
Melastomataceae (9/107) |
Calceolaria (Scrophulariaceae) (65) |
Gentianaceae (4/93) |
Espeletia s.s. (Asteraceae) (61) |
Ericaceae (16/79) |
(Espeletia s.l. =123) |
Bromeliaceae (6/78) |
Lupinus (Fabaceae) (56)* |
Rosaceae (10/77) |
Valeriana (Valerianaceae) (54) |
Fabaceaea (9/76) |
Hypericum (Clusiaceae) (54) |
Brassicaceae (13/71) |
Miconia (Melastomataceae) (54) |
Cyperaceae (8/70) |
Gentianella (Gentianaceae) (48) |
Apiaceae (15/61) |
Puya (Bromeliaceae) (48) |
Solanaceae (8/58) |
Gynoxys (Asteraceae) (46) |
Clusiaceae (2/56) |
Baccharis (Asteraceae) (45) |
|
Draba (Brassicaceae) (45) |
|
Geranium (Geraniaceae) (43) |
|
Solanum (Solanaceae) (43) |
*Rupert Barneby, who studied
Lupinus, felt there are only about 15 species in the genus. If this is
true the overall family number drops to ca. 35 species. |
|
Table VII.
Vascular plant genera endemic (or nearly so) to the páramo. Those marked with
an asterisk (*) are monotypic. |
|
VASCULAR PLANTS |
|
Apiaceae |
Laestadia |
Cotopaxia |
Paramiflos* |
Perissicoelum |
Raouliopsis |
Asteraceae |
Westoniella |
Aphanactus |
Campanulaceae |
Ascidiogyne |
Lysipomia |
Blakiella* |
Melastomataceae |
Chrysactinium |
Castratella |
Coespeletia |
Scrophulariaceae |
Espeletia |
Aragoa |
Espeletiopsis |
|
Floscaldasia* |
FERNS |
Flosmutisia* |
Pteridaceae |
Freya* |
Jamesonia |
Hinterhubera |
Nephopteris* |
Jalcophila |
|
|
General Phytogeography and Origins.
Frequently, the overall floristic and phytogeographical relationships of
the páramo flora have been discussed and compared with the Mexican
and Guatemalan alpine floras (Beaman, 1965; González, 1986;
Islebe & Cleef, 1995; Rzedowski, 1978), the tepuis of the Guayana
Highlands (Cleef et al., 1993; Riina, 1996), the lowland savannas
of South America in general (Cleef et al., 1993), the puna flora (Baumann,
1988; Quintanilla P., 1983b), the subantarctic flora (Cleef, 1978,
1980a), and the world's other high elevation tropical floras such as in
Africa and Malesia (Smith & Cleef, 1988). In common with all
other high elevation tropical floras, the páramo flora is predominantly
of temperate zone origin at the generic level; but in contrast, the
páramo flora is the richest overall and has the largest actual number
of genera and endemic elements (Smith & Cleef, 1988). Future
research efforts are needed to see if the previous comparisons, based mostly
on a restricted data-set from the páramos of the Colombian Cordillera
Oriental, are representative for the páramo flora as a whole.
In an effort to understand the origins
of the páramo flora, recent phytogeographical studies have followed
Cleef (1979a) in assigning each genus to one of seven geographical floristic
elements: páramo, other neotropical, widespread tropical,
Holarctic, Austral-Antarctic, widespread temperate, and cosmopolitan (see
Table VIII). In the
Colombian Cordillera Oriental, the most comprehensively
studied páramo region to date, Cleef (1979a, 1980c) and Van der
Hammen and Cleef (1986) found that the páramo genera of vascular
plants are about 50% tropical in origin and 50% temperate. Within
the tropical element, strictly neotropical genera are the most strongly
represented. Within the temperate element, the widespread genera
are most strongly represented (about 20% of the genera), while the Austral-Antarctic
and Holarctic elements are each represented by about 10%. See also
Sturm and Rangel Ch. (1985), who studied the phytogeography of the 130
most important species of the Colombian páramo flora as a whole.
In the páramo flora of the Cordillera
de Talamanca (Costa Rica), Cleef and Chaverri P. (1992) found that of the
páramo genera of vascular plants about 36% are tropical in origin
and 64% temperate. Within the tropical and temperate elements of
Costa Rica, strictly neotropical genera and widespread genera, respectively,
are the most strongly represented, as they are in the Colombian Cordillera
Oriental. Although the Costa Rican páramo flora shares about
95% of its vascular genera with the Andes, the larger proportion of the
temperate component in Costa Rica, with genera such as Garrya (Garryaceae),
Arctostaphylos (Ericaceae), Mahonia (Berberidacee), and Romanschulzia
(Brassicaceae), was attributed to the more northern geographical position
of the country. [Note: The genera Helianthemum (Cistaceae)
and Smilacina (Liliaceae) have also been reported from the páramos
of Costa Rica by Cleef and Chaverri P. (1992), but I have not seen any
herbarium specimens unequivocally from páramo and, therefore, have
not included them in this book.]
Ricardi S. et al. (1997) studied the
superpáramo (above 4000 m) in Venezuela and compared it with other
high elevation floras along the entire length of the Andes from Costa Rica
to Patagonia. They found that 34% of the genera of vascular plants
are tropical in origin and 66% temperate, with the greatest similarity
to that of Colombia and next to Ecuador.
In northern Ecuador, León Yánez (1993) found that
of the genera of vascular plants 45% are of tropical origin and 55% of
temperate. In the southern páramos of Ecuador, Ramsay (1992)
found fewer families and genera than in Colombia, with about 33% of the
genera of vascular plants of tropical origin and 67% temperate. He
attributed this to the lower humidity and more extreme cold temperatures
of Ecuadorean páramos. Ramsay (1992) also compared the Ecuadorean
páramo flora to the puna of Peru and the mountains of East Africa
and New Guinea (see also Balslev, 1988; Mena V., 1984 and Mena V.
& Balslev, 1986). There are, unfortunately, no similar phytogeographical
studies from the páramos (jalca) of northern Peru.
Monasterio (1980b) looked at the entire
páramo region and found the following trend: the jalca and
Ecuadorean páramos consist mainly of tussock grasses with genera
from extra-tropical regions, the Venezuelan páramos are dominated
by rosettes, and the páramos of Colombia show equal importance of
grasses and rosettes.
For additional studies that include
phytogeographical analyses, see Ahti (1992, for the lichen family Cladoniaceae),
Becker (1988), Cabrera (1957), Cleef (1981b), Duque N. (1987), Jørgensen
and Ulloa U. (1994), Keating (1995), Lozano C. and Rangel Ch. (1989), Rangel
Ch. (1991b, 1995a), Rangel Ch. and Garzón (1995, 1997), Sipman (1992,
for Colombian lichens), Tirado M. and Ricardi S. (1997), Vargas Ulate &
Sánchez G. (Unpublished).
Table VIII.
Percentage of vascular plant genera in each phytogeographical element in the
Neotropical páramos. |
Geographical Element |
Percentage of Vascular Plant Genera |
|
CRa |
VEb |
CO-1c |
CO-2d |
EC-1e |
EC-2f |
|
Páramo |
4 |
7 |
7 |
8 |
4 |
9 |
Other Neotropical |
25 |
24 |
34 |
30 |
32 |
21 |
Wide Tropical |
7 |
3 |
10 |
28 |
10 |
3 |
Holarctic |
15 |
13 |
11 |
12 |
10 |
14 |
Austral-Antarctic |
14 |
7 |
9 |
5 |
10 |
10 |
Wide Temperate |
24 |
39 |
20 |
7 |
26 |
26 |
Cosmopolitan |
11 |
7 |
8 |
10 |
9 |
17 |
|
aCordillera
de Talamanca, Costa Rica (Cleef & Chaverri P., 1992); bbased
on data from the superpáramo of Páramo de Piedras Blancas, Venezuela
(Ricardi S. et al., 1997); cbased on ca. 600 relevés from all
parts of the páramo throughout the Cordillera Oriental, Colombia (Cleef,
1979a); dbased on the 130 most important species of the Colombian
páramo flora as a whole (Sturm & Rangel Ch., 1985);
ePáramo de Guamaní, Ecuador (León Y., 1993);
fbased on 192 quadrats in zonal vegetation from 12 scattered
páramos in Ecuador (Ramsay, 1992). |
Fauna
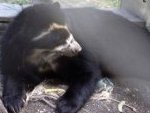 Spectacled bear |
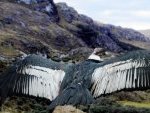 Andean condor |
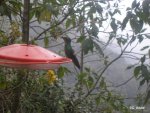 Hummingbirds |
Páramo grass- and shrublands support
a number of native animal species. It is not the purpose of this
book to discuss the general fauna of the páramos, but some of the
larger, more common, and conspicuous animals are mentioned here.
Mammals in the páramo include
the puma (Felis concolor), spectacled bear or "oso de anteojos" (Tremarctos ornatus), white-tailed deer (Odocoileus virginianus),
mountain tapir or "danta" (Tapirus pinchaque), rabbit (Sylvilagus
brasiliensis), guinea pig (Cavia porcellus), Andean fox (Duscicyon
culpaes), mountain coatí (Nasuella olivaceae), long-tailed
weasel (Mustela frenata), shrew (Cryptotis spp.), rat opossum
(Caenolestes spp.), and various small rodents. Rabbit feces
are extremely common in the grass páramo, especially in open areas
between tussocks.
Stotz et al. (1996) list 69 species
of birds as "total users" of the páramo habitat, with 41 species
making it their primary habitat and 16 as indicator species. Some
of the better known páramo birds include: vultures such as
the Andean condor (Vultur gryphus) and the turkey buzzard (Cathartes
aura), eagle, hawk, and falcon (Phalcoboenus carunculatus, Buteo
spp., Geranoaetus spp., Falco sparverius), owl (Bubo virginianus
and Asio spp.), hummingbird or "colibrí" (family Trochilidae:
e.g., Oxypogon guerinii and Patagona gigas), duck (Anas
spp.), and the rufous-fronted parakeet (Bolborhynchus ferrugineifrons).
See F. Vuilleumier (1986) for a discussion of the origins of the high Andean
bird fauna.
Native páramo fish species are
few, but rainbow trout or "trucha" (Oncorhynchus mykiss) have been
introduced to many lakes and streams for food and sport.
The herpetofauna has been relatively
well studied (see especially Duellman, 1979b) and includes amphibians such
as the salamander (Bolitoglossa spp.), frog and toad (Eleutherodactylus
spp., Hyla spp., Atelopus spp.), and reptiles such as the
lizard and cameleon (Stenocercus spp., Phenacosaurus spp.,
Proctoporus spp.).
Invertebrates are less conspicuous and
found mostly in the subpáramo. These include lepidopterans
(Descimon, 1986) and other insects such as grasshoppers, cockroaches, beetles,
and flies; molluscs such as a few snails and slugs; and earthworms.
Arthropods and other microfauna (e.g., mites and springtails) are rarely
seen, but are abundant (Sturm, 1978, 1983, 1990, 1994a; van Velez, 1992).
A number of the larger páramo
mammals, as well as the Andean condor,
have been extensively hunted by man and their numbers are now very low.
Hummingbirds, bees, and flies seem to be important pollinators in
the páramo (see, for example, Berry & Calvo, 1994; Brand
Prada, 1994b). Birds, rabbits, and guinea pigs (as well as wind, water,
and gravitation) are important agents of dispersal (Frantzen & Bouman,
1989; Graf Bock, 1984; Simpson & Todzia, 1990).
Additional references about the páramo
fauna include Aagaard (1982), Adams (1973), Amat García (1987, 1991a,b),
Arias Lemos (1989), Aristide U. (1969), Barnett and Gordon (1985), Barrientos
and Monge-Nájera (1955), Bernal C. (1985), Bernal C. & Figueroa
(1980), Brand Prada (1994a), Cadena G. and Malagón (1994), Chapman
(1917, 1926), Davis et al. (1997), Del Llano (1990), Duellman (1979a, 1988),
Fjeldså and Krabbe (1990), Garay (1981), E. Gaviria (1991), S. Gaviria
(1989), Grabandt (1983), Hoffstetter (1986), Hoyos (1991), INDERENA (1984),
Janzen et al. (1976), Lynch (1986), Mani (1962), Mora O. and Sturm (1994a),
Phelps and Phelps (1958, 1963), Rangel Ch. and Bernal (1980), Reig (1986),
Righi (1995), Rivero (1979), Sturm (1984a), Sturm and Rangel Ch. (1978),
F. Vuilleumier (1980), Vuilleumier & Ewert, (1978), Wolf and Gill (1986),
and Yerena (1994).
Human Influence
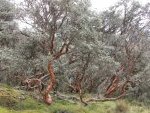 Polylepis forest |
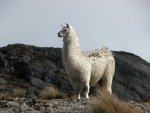 Llama |
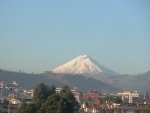 Quito |
There have been settlements in the highlands
of the Andes for perhaps 15,000 years or more (Eckholm, 1975; Little,
1981). Man's influence there has been profound, with the result that
nearly 90-95% of the forests of the northern Andes have been cleared (Henderson
et al., 1991). Exploitation of the high elevation puna ecosystem
of the central and southern Andes of Peru, Bolivia, and northern Argentina
occurred in the pre-hispanic period and has been relatively well documented
(see for example, Baker & Little, 1976; Brush, 1976, 1982;
Ellenberg, 1979). Intensive land use in the páramos of the
northern Andes has been a more recent phenomenon and needs further study
(Hess, 1990). Did similar changes of habitat destruction and alteration
take place in the essentially unpopulated high elevation páramo
areas as it did in the puna or in the lower montane regions of the Andes?
One of the most frequently discussed questions about the historical development
of highland regions of the northern Andes is whether the páramo
that we see today, specifically the grass páramo, is a natural ecosystem
or to what extent did man create it following his need to provide more
pasture and agricultural land?
Ellenberg (1979) championed the theory
that the climax vegetation of the tropical Andes was forest and that man,
largely by means of fire, has been responsible for nearly all the large
open areas (Becker, 1988; Fjeldså, 1992; Lægaard,
1986, 1992). Other authors feel that both Polylepis forest and some form of páramo grassland
have always existed as independent formations, and have expanded and
contracted through time in response to climatic changes (Cleef, pers.
comm.; Lauer, 1981; Troll, 1959; Van der Hammen &
Cleef, 1986; Walter & Medina, 1969a,b).
Charcoal fragments in core sediment
samples reveal that fires have occurred in páramo since Holocene
times (Horn, 1989c; Horn & Sanford, 1992; Salomons, 1986).
Those fires may have been ignited by natural sources (i.e., lightning or
volcanic activity) or by man (deliberate or accidental), but usually their
exact origins are unknown. Verweij (1995) has stated that fires caused
by natural sources occur less than once in 1000 years. Published
records of lightning-set páramo fires have not been found, although
Horn (1989c) and Young (pers. comm.) have mentioned lightning strikes in
Chirripó páramo (Costa Rica) and the Río Abiseo area
(northern Peru), respectively. In studies of the Costa Rican páramos,
Horn (1989c, 1993, In press; Horn & Sanford, 1992) has correlated
modern and prehistoric fires together with pollen analyses of sediment
cores. She has concluded that in the Chirripó highlands páramo
communities are the "natural" vegetation and that modern pollen assemblages
of individual taxa have not differed greatly from those developed since
deglaciation some 10,000 years ago. She has also found that fires
have been a part of Chirripó páramos throughout the Holocene,
but have not "carved páramo from forest."
Without doubt man has had major impact
upon the origin and subsequent spread of grasslands throughout the Andes,
and perhaps he has been the single most important reason why grass páramo
exists today, where shrub/tree woodlands of Polylepis, Buddleja,
and Gynoxys may have once dominated. It is unlikely, however,
that we will ever be able to say with confidence what percentage of today's
páramo has anthropogenic origins. Whatever the outcome of
this discussion, the facts remain that grass páramo currently exists,
covers large expanses of the high elevation Andes, and has great ecological
and economical importance.
The following discussion summarizes
what we do know about the history of man's presence in the páramos
of the high Andes and the effect he has had on them. Although many
of the references and quotes given below are specific to one country, I
am certain that similar events have occurred in all of the north Andean
countries including Venezuela, Colombia, Ecuador, and probably by extension
to Costa Rica and Panama. The situation in southern Ecuador and northern
Peru is less clear, however, and may be quite different due to its overall
drier climate and proximity to the larger pre-Columbian civilizations that
occupied high elevations to a much greater extent in the central Andes
than in the northern Andes. For example, the intense grazing pressure
may have occurred earlier in Peru because of the presence of native camelids
and their herding.
The Pre-Columbian Period.
It is assumed that the Venezuelan páramos have been exploited for
more than 1400 years (López del Pozo, 1992). They have been
occupied for at least 500 years, although there were no permanent settlements
until colonial times (Wagner, 1978, 1979, 1988). The páramos
were used, however, as corridors, in rituals, or as hunting areas (Clarac,
1981, 1985; Rojas, 1985). According to Salgado-Labouriau (1976)
and Monasterio (1980d), pre-Columbian agriculture was present in Venezuela
up to 3000 m, the lower edge of the páramo, but it was concentrated
below 2000 m and the cultures that developed in or near páramo were
dedicated to agriculture and hunting/gathering (Molinillo & Monasterio,
1997). In Colombia, the pollen record shows that human activity in
the páramos around Bogotá began about 800 years ago (Van
der Hammen, 1968). The Colombian páramos were mostly uninhabited,
however, although they too were used for the cultivation of some tuber
crops and as a crop-storage area (Langebaeck, 1988; López
del Pozo, 1992). The highest mountains and páramos were mostly
considered sacred, often with mystical qualities, and their main use was
for religious purposes and to bury the prominent dead (G. Correal U., cited
in Cleef, 1981b; Reichel-Dolmatoff, 1982; Zambrano, 1993).
The Colonial and Independence Periods.
Europeans settled the New World in the 16th century. They introduced
exotic animals that were totally new to the northern Andes, viz., cows,
sheep, goats, horses, and donkeys, which added to the herds of native camelids
(llama, alpaca, and vicuña) in Peru and further south.
[Llamas and alpacas have only recently been introduced into the Ecuadorean
páramo (Hervas Ordoñez, 1994).] Exotic plants were also
introduced by Europeans for cultivation at the cooler high elevations,
especially the grains wheat and barley, but also vegetable crops such as
broad or faba beans, lentils, peas, carrots, radishes, onions, and garlic.
These new plants added to the native grain quinoa and tuber crops such
as potato.
Early colonists (including pre-Columbian
man) founded their villages and towns primarily in the cooler inter-andean
valleys along rivers, where their crops were grown in the fertile floodplains
(corn) and along the adjacent upland slopes (potatoes and grains).
Cattle reached the Andes of Venezuela and Colombia in the late 1500s, but
probably were not introduced into the páramos until the early 1700s,
and then only to ca. 3000 m (Vila, 1962; Wagner, 1967). The
cattle foraged in the páramo grasslands above the forest, several
hours or days walk from their homes. As herds of cattle grew in numbers,
fires were periodically set to burn-off the dead material from the páramo
and provide fresh new grass for the cattle. With time the páramo
became overgrazed and excessively burned. Wood was also cut from
nearby forests, mostly for fuel and as building material (including road-building).
Gradually, the need arose to cut and burn the forest immediately below
the páramo to provide additional new grasslands for pasturing and
arable land for crops. Sediment cores from Laguna Victoria, Venezuela
(3250 m), show that at about this same time large amounts of pollen of
Rumex acetosella, an herb introduced with wheat and potatoes, as
well as a drastic drop in tree pollen, suggesting a massive deforestation
of the upper Andean forest (Salgado-Labouriau & Schubert, 1977).
The numbers of cattle continued to rise
steadily during the 1700s. In the 1800s, during the wars of independence
and civil strife in Venezuela (and possibly throughout Colombia, Ecuador,
and Peru), the numbers of cattle oscillated, sometimes decreasing by almost
95%, although they again reached the pre-independence levels by ca. 1847
(Brito, 1972; Pérez, 1992a, In press; Rouse, 1977;
Vila, 1962, 1978).
Thus it would seem that in the early
1700s the páramos of the northern Andes were still not utilized
to the extent of the altiplano or puna to the south (Brush, 1982).
However, during the late Colonial period and into the Independence period,
agriculture and cattle raising became common (Velázquez, 1986) and
the range of páramo-like habitats probably expanded.
The Modern Period. During
the 20th century, man's impact on páramo has been a story driven
by demographics, i.e., the numbers, density, and distribution of populations.
As population densities in the interandean valleys
increased, people began to colonize new areas in order to satisfy their
needs (Bernsen, 1991; Brush, 1976, 1982; Ellenberg, 1979;
Hess, 1990; Hofstede, 1995c; López del Pozo, 1992;
Molinillo & Monasterio, 1997; Monasterio, 1980d; Parsons,
1982; Pérez, 1992a). The annual population growth rates,
previously over 3% in Venezuela, Colombia, Ecuador, and Peru (Little, 1981)
and currently 2.6-2.9% (World Population Data Sheet 1997 from the Population
Reference Bureau), are still the highest in South America. The Colombian
population, for example, increased from 3 million to 12 million between
1900 and 1950, rose to 26 million in 1975, to 33 million in 1990, and is
estimated that it will reach 49 million in 2025; the Venezuelan population
increased from 5 million to 20 million between 1950 and 1990, rose to 22
million in 1995, and is estimated that it will reach 35 million in 2025;
while the Ecuadorean population increased from 3 million to 11 million
between 1950 and 1990, rose to 12 million in 1995, and is estimated that
it will reach 18 million in 2025 (World Resources 1996-97).
Because of land and agrarian reform
policies during the 1960s and 1970s, most parts of Colombia, Venezuela,
Ecuador, and northern Peru have experienced two recent tendencies:
the upward movement of agriculture into the páramo belt between 3000 m and 4000 m,
and the intensification of animal production in the lower páramo
belts (Baruch, 1979; Becker, 1988; Bernsen,
1991; Ferwerda, 1987; Hess, 1990; López-Zent,
1993; Monasterio, 1980d; Pérez, 1992b; Verweij,
1995; Verweij & Beekman, 1995). In Venezuela, Monasterio
(1980d) reported that since the 1960s, the highest limit of cultivation
(in Páramo Piedras Blancas, ca. 4200 m) has increased due to the
introduction of frost-resistent varieties of potato, such as the "papa
negra."
The last 20-25 years have also seen,
however, a regional growth in urbanization, with farmers abandoning the
countryside to cities such as Mérida, Bogotá, Nariño,
Quito, Cajamarca, etc. This has resulted in greater demand and increased
pressure upon fewer farmers throughout the Andes to cultivate new land
to produce more food. As natural soil fertility decreased in the
páramo, usage of chemical fertilizers, insecticides, pesticides,
and fungicides increased (Bernsen, 1991; Ferwerda, 1987; López-Zent,
1993), followed by soil contamination. For example, in the crop belt
of the Venezuelan páramos (3000-4000 m), the traditional land-use
system of short periods of crop production (1-4 years) alternating with
fallows of 7-20 years, which allows long-term recuperation of nutrients
with negligible erosion rates and maintenance of high levels of soil organic
matter, has been interrupted by massive use of chemical fertilizers and
a market-oriented agricultural system. Some of the consequences have
been "greater incidence of pest outbreaks, soil exhaustion, a possible
decrease in soil organic matter, and intensification of erosion" (L. Sarmiento
M. et al., 1990, 1993). According to Ferwerda (1987), more than 70
years are needed for regeneration of páramo bunch-grasslands after
potato cultivation. Along with the upward movement of agriculture
and increased livestock production have come the continued lowering of
the forest line due to wood cutting to make more room for crop production
and pastureland (Kok et al., 1995). The timber is used (locally and
regionally) for building, fence-posts, fuel, etc. Fuelwood collection,
mainly for cooking purposes, also removes shrubby vegetation from the páramo
proper (Becker, 1988). Verweij (1995) estimated that an average family
of seven persons uses about 20 kg of fuelwood every day, which corresponds
to a cleared area of one hectare per year.
Other recent developments that have
led to additional páramo disturbance include construction of aqueducts,
drainage systems, and roads (Young, 1994), off-road vehicles become more
common (Pérez, 1991b), mining (especially of sand, gravel, and peat),
and afforestation projects (Rangel Ch., 1989; pers. observ.).
The impact of plantations of exotic tree species (especially
Pinus
radiata and P. patula) on the development of soils and vegetation
placed in or near (extensively grazed) páramo has recently been
described by Hofstede (1997). Even tourists are now becoming a problem
in some areas (Horn, 1986; Urgilés Sánchez, 1990).
Impact of Burning and Grazing
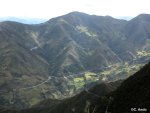 Burning |
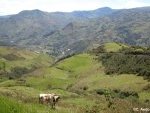 Grazing |
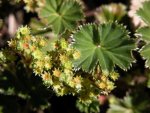 Lachemilla orbiculata |
Fire is used in the páramo for
primarily two reasons. First, it is a means to clear the upper limits
of forest, thus providing open grasslands for grazing of livestock and
new land for cultivation of crops. Second, because forage quality
is poor and up to 80% of the above ground biomass of the tussock- or bunchgrass
growth form is dead material (Cardozo & Schnetter, 1976), farmers burn-off
the old or dead non-palatable parts to provide new, more nutrient-rich
growth for cattle. Ramsay and Oxley (1997) say that it is quite difficult
to estimate the ultimate effects of fire upon páramo vegetation
and its species diversity, since fire has been so much a part of páramo
history. Nevertheless, burning and grazing go hand-in-hand in most
high elevation areas of the northern Andes; seemingly they are the
only applied "management practice." However, is fire necessarily
bad for páramo in view of the fact that it has been part of páramo
history since at least Holocene times? And what are the actual effects
of fire and grazing upon páramo? How do the continuous and
repetitive practices of burning and grazing combine to affect species composition,
nutrient and soil-water content, biomass and productivity, decomposition,
and short-term and long-term stability of the páramo ecosystem?
The following discussion outlines what is currently known about the role
and impact of fire on the páramo ecosystem.
Four recent studies about the combined
effects of burning and grazing on the páramo ecosystem contribute
new insight and provide valuable basic information that is necessary for
future management programs (Hofstede, 1995c; Keating, 1995;
Ramsay, 1992; Verweij, 1995). In the following section, I have
drawn heavily from the excellent dissertation of Robert Hofstede (1995c),
who studied the ecological impacts of burning and grazing on biomass, nutrient
status of vegetation and soil, and hydrology, in the Los Nevados National
Park (Cordillera Central, Colombia). In a complimentary study, Pita
Verweij (1995) focused on the impacts of management on vegetation development
and landscape ecology in the same national park. In addition, Paul
Ramsay (1992) and Philip Keating (1995) studied páramo vegetation
in Ecuador, including community ecology, dynamics, and productivity.
Some of their main conclusions are summarized in the following paragraphs.
Hofstede (1995c), Lægaard (1992),
Ramsay (1992), and Verweij (1995) all conclude that natural páramo
vegetation is to a certain extent tolerant to the managament practices
of burning and grazing, and that fire does offer some short-term advantages
to the cattle-farmer (over-all palatability, initial productivity).
It is not, however, a wise long-term management tool, because when the
burning frequency is more than once per decade (Verweij, 1995) and grazing
intensity increase, stress on the vegetation is too great, effects are
mostly negative, and there is degradation of the ecosystem, including a
loss in species diversity. Unfortunately, it has been shown that
farmers will choose the short-term perspective, i.e., rapid post-fire regeneration
of palatable forage for maximum livestock production at the expense of
long-term degradation of the vegetation and soil (Hofstede, 1995;
Ramsay, 1992; Verweij, 1995).
Burning and grazing affect the páramo
ecosystem most importantly in the following three ways:
Vegetation Structure, Composition,
and Species Diversity. Despite their many adaptations, rosette
plants (especially members of the Espeletiinae) and tussocks or bunchgrasses
are some of the most susceptible species to burning and grazing in the
páramo. In Venezuela, A. P. Smith (1981) found that 55% of
the adult Espeletia schultzii (Asteraceae: Espeletiinae) plants
could be killed by fire in a dry season, while in Colombia, Verweij and
Kok (1992, 1995) found over 80% mortality in juveniles of E. hartwegiana
immediately after fire. Verweij and Kok (1992) also found that grazing
had a major impact on adult mortality of E. hartwegiana in Colombia,
and Pérez (1992a) found that cattle browsing could cause a decrease
of 37% in rosette cover of Coespeletia timotensis (Asteraceae: Espeletiinae)
in Venezuela. In southern Ecuador, Keating (1995) found that burning
and cutting reduced the number of rare species and the relative frequency
of shrub species. In the Buenavista subpáramos of Costa Rica,
Horn (1997, In press) found that 90-99% of the dominant bamboo, Chusquea
subtessellata and the ericaceous shrub Vaccinium consanguineum resprouted
following fire, while the second most common woody species, the shrub Hypericum
irazuense (Clusiaceae), suffered 94% mortality. This display
of fire sensitivity in woody species certainly affects species composition
and may be the reason for the present dominance of the bamboo. For
tussocks and bunchgrasses, burning and subsequent grazing also lead to
fragmentation because of trampling by livestock after the fire. Continued
grazing or overgrazing leads to replacement of the tussocks and bunchgrasses
by short-grass communities (Agrostis, Festuca, Paspalum), rosaceous
species (Acaena and
Lachemilla orbiculata), and species of
low or matted herbs such as Eryngium humile (Apiaceae), Bidens
and Hypochaeris (Asteraceae). These plants are better adapted to
cattle grazing and to more exposure of bare ground.
Alien species, which are frequently found in these communities, are indicators
of human and grazing (with some fire) disturbance; these include
(among others) Achillea millefolium, Gamochaeta americanum (formerly
Gnaphalium americanum), Stevia lucida, and Taraxacum officinale
(Asteraceae), Arenaria serpens, Cerastium arvense and Stellaria
media (Caryophyllaceae), Trifolium repens and Lupinus
spp. (Fabaceae), Geranium multipartitum (Geraniaceae), Juncus
effusus (Juncaceae), Oxalis spp. (Oxalidaceae), Anthoxanthum
odoratum, Dactylis glomerata, Digitalis purpurea, Poa annua and Holcus
lanatus (Poaceae), Rumex acetosella (Polygonaceae), and the
fern Pteridium aquilinum (Dennstaedtiaceae) (Cleef, 1981b;
Cleef & Rangel Ch., 1984; Ewel et al., 1976; Hofstede,
1997; Hofstede, Chilito P. & Sandoval S., 1995; Hofstede
et al., 1995; Keating, 1995; Ramsay, 1992; Rangel Ch.,
1989; Salamanca, 1991; A. P. Smith, 1974a; Verweij &
Budde, 1992; Verweij & Kok, 1995).
Based on studies of small-scale dynamics
within several páramos in Ecuador, Ramsay (1992) generalized that:
a) it is mainly vegetation structure that determines fire temperature:
the highest temperatures (up to 600°C) are produced in the tussock
canopy while the lowest (ca. 65°C) are found within the tussock base
and just beneath the soil surface; b) the main form of recovery from
fire is regeneration by vegetative reproduction of below ground plant parts;
c) the degree of survival of intertussock species depends upon the severity
of the fire, which is largely a function of the time interval since the
last burning; d) survival following fire does not guarantee a plant's
persistence in the community, since both tussock grasses and intertussock
species show significant mortality rates in the months following fire;
e) recovery at higher altitudes is slower; f) burning may cause the
patterns of community development to become cyclical; g) some species
that quickly colonize bare ground by seed may persist, but as the vegetation
matures most are killed by competition; and h) establishment within
tussocks after burning is the preferred method of some species, such as
Lupinus cf. pubescens.
Minimum fire recovery periods, i.e.,
the period of post-fire growth required to generate enough fuel to carry
a subsequent fire (Verweij, 1995), of 3-10 years have been reported depending
on the vegetation structure (Horn, 1990, 1991; Janzen, 1973;
Ramsay, 1992; Ramsay & Oxley, 1996; van Groen, 1987;
Verweij, 1995). However, the frequency of burning is typically every
2-4 years (Ramsay & Oxley, 1996), and in practice, fire is applied
too frequently to allow full bunchgrass recovery (Verweij, 1995).
Minimum fire recovery periods do not mean that the vegetation will have
recovered completely, however, because species composition and distribution
will change and more bare spots will be present (Hofstede, 1995c;
Horn, 1989; Janzen, 1973; Miller & Silander, 1991;
Verweij, 1995; Williamson et al., 1986). Furthermore, since
grasses have high relative growth rates, and burning and grazing stimulate
tillering, they are favored over woody species by high fire frequencies
(Horn, 1991; Janzen, 1973; Ramsay, 1992; Ramsay &
Oxley, 1996; Verweij, 1995; Williamson et al., 1986).
Verweij (1995) concluded that more than 10 years are needed before the
average number of vascular plant taxa stabilizes back to pre-fire levels,
implying also that fire frequency may influence species richness in the
vegetation.
In summary, it would appear that occasional
disturbances over short intervals may allow for relatively rapid recovery
of community structure and composition, but that repeated burning, combined
burning and grazing, or severe disturbances at intervals of even 2-4 years
is too great to allow complete recovery of páramo vegetation and
some species are lost.
Soil Structure and Water-storage Capacity.
The páramo belt is an indispensable source of water for drinking
and irrigation for a majority of the people in Venezuela, Colombia, and
Ecuador. In its natural, undisturbed condition, páramo acts
as a sponge, where the excess water is slowly but constantly returned to
the ecosystems of lower elevations (Gómez Molina & Little, 1981).
Natural páramo shrub vegetation holds up to 12 times more water
than a disturbed grass community (Schnetter et al., 1976), while water
retention in soils of the páramo surpasses 200% of its own dry weight
(Cañadas Cruz, 1983). Hofstede and Sevink (1995) showed that
undisturbed páramo has a larger water-storage capacity than burned
and grazed páramo, and that a continuously wet plant layer is important
for maintaining the large soil storage for dryer periods. With grazing
and burning, however, vegetation cover disappears and is replaced by a
low growing, ground cover that cannot hold as much moisture since the páramo
soils are more compressed, dryer, less acidic, and somewhat less organic
(Hofstede, 1995c). Verweij and Budde (1992) and Verweij (1995) also
found that during the first 1.5-5 years following fire the percentage of
bare soil increased over 10%, depending on the vegetation structure and
grazing intensity. Meanwhile vegetation is trampled and compacted,
and the turf is broken-up by cattle (Grubb, 1970; Pérez, 1993a),
thus favoring erosion, loss of soil, and micro-terracing, especially on
slopes (Flores Ochoa, 1979; Pérez, 1992b, In press;
Troll, 1973).
Decomposition, Nutrients and Productivity.
Hofstede (1995) and Verweij (1995) have shown that grazing and especially
burning practices result in faster decomposition and mineralization due
to dryer soils and higher maximum soil temperatures, but this does not
necessarily lead to higher nutrient contents of soils and plant tissues.
On a short-term basis, mineralized nutrients may become available for immediate
plant regrowth, but generally they are very quickly unavailable in the
soil due to immobilization especially of phosphorus, volatilization especially
of nitrogen and sulphur, or less importantly to erosion or leaching.
Therefore, the vegetation remains nutrient poor. The change in vegetation
structure and composition eventually leads to less tussocks, which may
have more tillers per unit area and a higher live material content, but
the overall lower stature and leaf elongation rates yield less productivity
(Hofstede, 1995). This low productivity combined with nutrient poor
vegetation results in poor quality cattle (Hofstede, 1995; Pels &
Verweij, 1992; Schmidt & Verweij, 1992; Verweij, 1995).
In summary, the general impact of increased
burning and grazing was summarized by Verweij (1995) as follows:
"the structure of the bunch grasslands becomes more open. Fire events
cause temporal changes in vegetation structure over the relatively short
time span of a few years. Without the influence of grazing, the vegetation
is able to recover and returns to the initial stucture of dense bunch grassland,
the floristic composition remaining largely unchanged. Under prolonged
grazing pressure, however, short matted grasses and forbs increase and
a gradual transformation into other communities occurs. Changes in
structure are of vital importance in relation to the water holding capacity
of both vegetation and soil. Hofstede & Sevink (1995) concluded
that water retention capacity is reduced in grazed and burned situations,
with an increased risk of drying especially in the drier seasons."
The Future of the Páramo Ecosystem
The páramo is rapidly becoming
a threatened ecosystem. With population size at its greatest in history
and steadily rising, pressure for greater food production and land use
has intensified and deterioration of the environment has inevitably followed.
Activities such as deforestation, road building, burning, agriculture,
and herding of animals have significantly altered this fragile ecosystem.
They have also caused native páramo plants and timberline forests
to disappear at an alarming rate, thereby allowing weedy species to dominate
some landscapes. Such activities also lead to accelerated erosion
on the slopes and flooding as the soils are not able to retain moisture
within their drainage basins. Furthermore, with the public's increased
desire for leisure time and a gradual awakening to the potential of the
páramo for recreation and tourism, we need to increase quickly our
efforts to study this ecosystem, not only to know what is there by means
of inventory and ecological analyses, but also to monitor the effects of
disturbance upon the vegetation and aquatic resources so that rational
management programs can be established to protect and preserve the páramo.
Why is the páramo important and
what needs to be done to maintain this ecosystem? Can we still turn
the tide of alteration and destruction? Must it be totally preserved
and protected? As has been discussed above, páramos are important
for scientific, ecological, and economic reasons -- all of which are inter-connected
and dependent upon each other. Some of these reasons are summarized
below and opinions are offered about what should be done.
Scientific Reasons. Páramos
occupy no more than 2% of the land area of the northern Andes in Venezuela,
Colombia, and Ecuador, and yet they are highly diverse biologically and
contain many plants that grow nowhere else in the world. Their geographical
isolation along the mountainous chain from Costa Rica to northern Peru,
along with their high diversity and endemism, combine to make them biogeographically
unique. Paleoecological studies indicate that páramo habitats
have undergone repeated expansion and contraction during the late Pliocene
and Pleistocene. These high elevation, cold-climate ecological islands
of endemism are surrounded by a sea of low elevation, warm tropical forest.
Therefore, the insular dispersal, age, origin, affinities, and evolutionary
patterns of the plant taxa, are open to detailed systematic and evolutionary
biogeographic studies.
Man's activities, however, are severely
limiting biodiversity in the páramo ecosystem. Basic facts
about the taxonomy, relationships, distribution, and ecological preferences
of most high elevation groups is very incomplete. We must know the
names of the taxa we are dealing with before we are able to compare them
or effectively communicate data about their ecology, evolution, etc. (Vuilleumier
& Monasterio, 1986). Therefore, it is appropriate and timely
that páramo plants be studied and a complete flora with keys, species
descriptions, and indications of geographical distribution be written.
Ecological Reasons.
Perhaps the single most important ecological function of páramo
is as a regulator of Andean hydrology. However, deterioration of
the páramo due to loss of vegetation cover, soil compaction, and
weakened water storage capacity, may increase soil loss, erosion, and flooding
and contribute to droughts during dry periods. Therefore, ecological
studies must also begin at the basic level, including additional short-
and long-term studies of the dynamics of páramo vegetation as it
affects landscape heterogeneity and plant species diversity, keeping in
mind the use of better management techniques that could lead to stabilization
of water supplies, increased productivity, and a subsequent lesser need
to cut more forest and exploit new areas of land (Ramsay, 1992; Verweij,
1995). Also needed is a better understanding of the dynamics of fire,
repeated fires, and recovery after fire in order to properly assess its
role as a tool in the management and conservation of páramo ecosystems.
It has also been noted that the páramo
is a very sensitive ecosystem and could provide an excellent area for additional
environmental studies of global and regional climatic changes, such as
the effects of global climate change (the greenhouse effect) and drought
or increased rain (brought on by El Niño) on plant species distributions
and adaptations.
Economic Reasons.
The major economic use of the páramo today is for agriculture and
livestock. But, as has been discussed above, the management practices
of burning, grazing, and increased use of chemicals emphasize only short-
not long-term usage. If the páramo ecosystem is going to remain
an important ecological and economic center, it must continue to be the
natural source of clean water, as well as a resource for agriculture and
grazing, new foods, ornamentals and medicines, and for recreation and tourism.
Páramo plants are contributors
of important genetic factors to high elevation or cool-habitat crops such
as the potato (Solanum tuberosum, Solanaceae) and the grain quinoa
(Chenopodium quinoa, Chenopodiaceae). Páramos are also
a source of potential new foods ("new" to non-Andean peoples) derived from
pre-Columbian
tuber crops such as "oca" or "íbias" (Oxalis tuberosa,
Oxalidaceae), "ulluco" or "olluco" (Ullucus tuberosus, Basellaceae),
and "añu", "mashua" or "cúbios" (Tropaeolum tuberosum,
Tropaeolaceae) (King, 1988; National Research Council, 1989;
Schjellerup, 1989). New ornamental plants such as Senecio niveo-aureus
(Asteraceae), and new medicines such as those derived from Oritrophium
spp. and Chuquiraga jussieui (Asteraceae), and Thamnolia vermicularis
(a lichen of uncertain affinity) have as yet unrealized potential (Aranguren
B. & Márquez, 1995; Aranguren B. et al. 1996; López-Zent,
1993; Paredes, 1962; Pérez, In press). Historically,
the páramo has been a source of traditional medicines for local
communities, and even today 56% (43 out of 77) of the medicinal plants
used by the Cogui, an indigenous people living in the Sierra Nevada de
Santa Marta (Colombia), come from the páramo (Carbonó de
la Hoz, 1987).
Although there are limitations, the
potential for ecotourism or for recreation in páramo regions, such
as fishing, hiking, and camping, has gone almost unnoticed (Black, 1983,
1988; Ramsay, 1992; Rangel Ch., 1989; Urgilés
Sánchez, 1990). The so-called "wilderness experience" can
draw many visitors, but as Hofstede (1995) has reminded us these same visitors
are "disappointed when meeting unnatural elements such as cattle, fences
and meadows. Both national and international tourists potentially
visiting the páramo are not directly interested in a high species
richness, a high water storage or a good soil protection, but their demand
is that the landscape is as original as possible: they simply enjoy
touching the snow and seeing an Espeletia. Nonetheless, the
ecological and hydrological sustainability benefits directly from the conservation
of an original landscape and vegetation structure... In the future,
it should be evaluated which yields more money: a productive cow
or a majestic Frailejón."
What can be done? Virtually any
extended use of the high elevation páramos, be it for science, economics
(including both resource production as well as extraction), or conservation,
will to some extent have an impact on species composition, vegetation structure,
biodiversity, and soil hydrology. It is only if all human activities
in páramos are ceased, that ecological and hydrological sustainability
will be assured. With the current socio-economic situation in the
Andes, however, the exclusion of all human activities from the páramo
is not realistic and agricultural production has to be accepted and respected
(Hofstede, 1995; Lauer, 1993; Verweij, 1995). Given the
scientific evidence at hand, it is relatively easy to identify the major
problems that have changed the páramo ecosystem, but what are the
practical alternatives? At this time, three areas in particular need
to looked at -- management practices, conservation efforts, and environmental
education.
Management. It seems generally
agreed upon that there is not any single management practice that addresses
all páramo functions; therefore, perhaps the challenge to
managers is to vary their programs in order to meet the demands of one
function in one area and those of another somewhere else (Hofstede, 1995;
Horn, In press; Pérez, In press; Ramsay, 1992;
Verweij, 1995; Young, 1997). While banning the use of burning
is advocated by some (e.g., Hofstede, 1995; Verweij, 1995), Horn
(In press) feels that fire management efforts need to be changed from the
prevention of all fires to the control of fire frequency thus recognizing
its place in the páramo landscape. Ramsay (1992) advocated
shifting agriculture and grazing to lower elevation zones, since it appeared
from the results of his productivity studies that lower altitude pastures
were "substantially under-productive." Rangel Ch. (1989) suggested
declaring all páramos as nationally protected areas, primarily to
protect the water resources; restricting use of all páramo
bodies of water, especially in the production of energy; avoiding
the exploitation of páramo biological resources and especially avoiding
grazing by sheep; the establishment of research centers to study
páramo; and using particular páramo areas for recreation
(with controls). Numerous smaller, but nonetheless important, changes
should also be considered for implementation wherever possible, such as:
prohibition of the cutting of natural vegetation and the collection of
fallen stems for fuel, use of fencing to exclude cattle or allow rotation
of grazing, and the exclusion of motorcycles and off-road vehicles from
the páramo (Pérez, In press).
If new management strategies are to
be considered, there needs to be strong coordination and cooperation between
scientists, local people, non-governmental institutions, and government
departments in the entire planning, design, and implementation process.
Becker (1988) has stressed that within indigenous communities there are
needs for alternative sources of income to alleviate the pressures of habitat
destruction for agricultural purposes (see also Verweij et al., In press),
better social organization with the promotion of traditional technologies,
such as terracing for soil conservation (Lauer, 1993; Nieto Cabrera
et al., 1997; L. Sarmiento M. et al., 1993), reforestation with useful
and native species (Brandbyge, 1984; Brandbyge & Holm-Nielsen,
1986; Lauer, 1993; L. Sarmiento M. et al., 1993), and integrated
agroforestry systems and controlled grazing and burning to allow regeneration
of native plants (Hofstede, 1995c; Ramsay & Oxley, 1996;
Verweij, 1995).
Conservation. Because of
their high biological diversity and their biogeographical, evolutionary,
ecological and economic significance, páramos are unique and the
species that have adapted to them will not grow elsewhere. These
facts, along with the realization that the páramo, like so many
other tropical ecosystems, is being altered by human activities, qualify
them as high priority areas for conservation. "Their preservation
in the face of these ever-expanding human activities, however, will depend
on their rational use" (Vuilleumier & Monasterio, 1986). The
farmer's aim in burning is to increase livestock productivity and this
practice may work in the short-term; however, the process of recovery
in páramo takes a long time and subsequent repeated burning and
grazing may cause long-term damage in complete contrast to the original
aim of the burning (Ramsay, 1992). Therefore conservation of the
páramo ecosystem, including watersheds and natural vegetation, is
imperative.
Until about 40 years ago there was little
direct action taken toward preservation of the páramo ecosystem.
Recently, however, páramos have been included within the boundaries
of many national parks, frequently with recognition as regulators of Andean
hydrology. National parks that include important stretches of páramo
are Chirripó (Costa Rica), Sierra Nevada de Mérida and Tamá
(Venezuela), Sierra Nevada de Santa Marta, Sierra Nevada de Cocuy, Sumapaz,
Chingaza, Los Nevados, and Puracé (Colombia), Cotopaxi, Sangay,
and Podocarpus (Ecuador), and the Río Abiseo watershed in northern
Peru. More representative páramo areas still need to be set
aside, however, as well as more efforts to increase the numbers of rangers
to enforce existing boundaries and park regulations.
Environmental Education.
Scientists have the responsibility to see that factual data from páramo
studies be presented to the general public, local authorities, and indigenous
communities, because if conservation and/or management goals are to be
achieved, public awareness and public support are essential. People
from large cities like Bogotá, Quito, Cuenca, and Mérida
flock to the páramos on weekends for the
natural beauty, the "wilderness experience" and for recreation, but at
the same time they often use branches from shrubs for their campfires
and leave litter behind (Urgilés Sánchez, 1990). We
must make the general public aware of the value of páramo,
which can also serve as an educational model in student field trips
and ecological tours. For example, placing signs along roads
through the parks that explain different aspects of the environment
are an excellent means of public education. If, as Horn (In press)
advocates, fire is recognized as having a place in the páramo landscape,
then park managers will also need to educate the general public as to the
role of fire in maintaining diversity of habitats and species.
The training of local students is also
critical for long-term maintenance of diversity and identification of critical
areas for protection and management (Balslev & Paz y Miño C.,
1991; Verweij, 1995). For logistic reasons, local scientists
can more easily undertake long-term surveys and their follow-ups.
Furthermore, many young scientists will become key government officials
or leaders in conservation groups in their countries, thus improving the
quality of decision-making about conservation and management issues.
Web page designed by Myriam Fica.
Contact Carmen Ulloa.
Top
|